Electric vehicles are transforming transportation, and at the core lies the electric vehicle batteries – a sophisticated energy storage system, not just a bigger car battery. Unlike gasoline tanks, these meticulously engineered powerhouses deliver power, range, and performance for a cleaner future.
This electric vehicle battery hub is your one-stop shop for EV battery knowledge. We’ll dissect different electric vehicle battery chemistries, explore their pros and cons, and delve into cutting-edge electric vehicle battery advancements pushing the boundaries of range, safety, and sustainability.
Table of Contents
- How Electric Vehicles Work
- Which Batteries Are Used in Electric Vehicles?
- Comparison of Batteries Used in Electrical Vehicles (Table)
- Factors to Consider When Choosing Electric Vehicle Batteries
- Electric Vehicle Batteries Recycling
- Final Thoughts
How Electric Vehicles Work
In an electric vehicle battery system, The battery pack serves as the primary energy storage system, storing electricity for the vehicle’s operation. Meanwhile, the AC-DC converter facilitates charging by converting incoming AC power from the EV Charger into DC for the battery.
The DC-AC converter transforms the direct current (DC) from the battery pack into alternating current (AC) to power the motor. The motor utilizes this electricity to generate rotational force, propelling the vehicle forward. The transmission adjusts the power from the motor, optimizing performance for various driving conditions. This power is then transferred to the wheels, which make contact with the road surface, enabling the vehicle to move.
Finally, the EV charger connects the vehicle to an external power supply, allowing for recharging of the battery pack. This integrated system forms the basis of how battery electric vehicles function, providing efficient and sustainable transportation solutions.
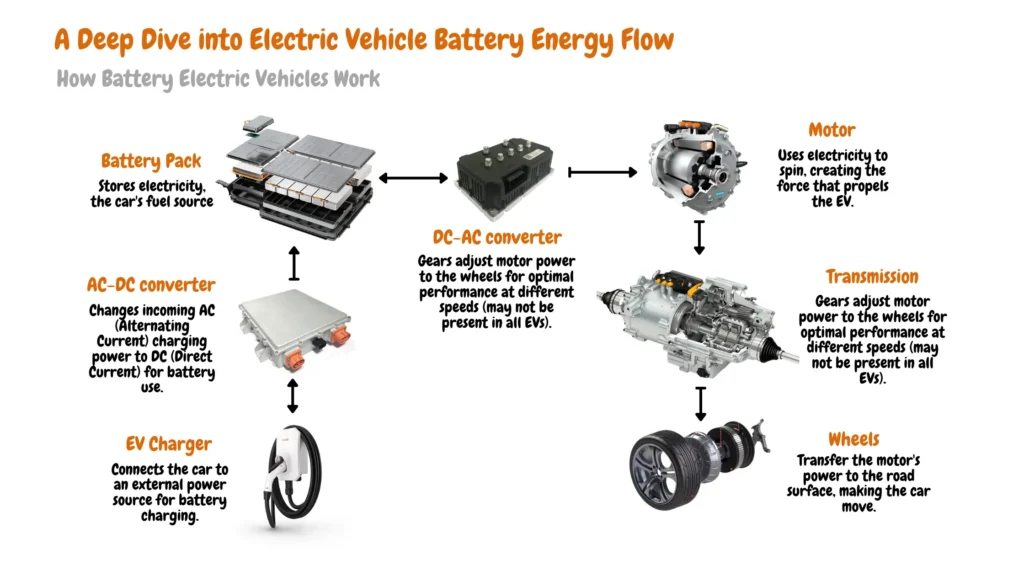
Which Batteries Are Used in Electric Vehicles?
Most electric vehicles (EVs) predominantly rely on Lithium-ion (Li-ion) batteries due to their optimal blend of energy density, weight, and charging efficiency. However, the EV industry is diversifying its battery choices beyond Li-ion, exploring alternatives like Lithium Iron Phosphate (LiFePO4 or LFP) Batteries, Lithium Nickel Manganese Cobalt Oxide (NMC) Batteries, Lithium Manganese Oxide (LMO) Batteries, Solid-State Batteries, and Sodium-Ion Batteries. Each type presents distinct advantages and drawbacks, contributing to ongoing research and development in EV battery technology.
Let’s delve into the most common battery types used in EVs today, along with their key characteristics and environmental considerations.
Lithium-Ion (Li-ion) Batteries
The current workhorse, Li-ion batteries offer a good balance of energy density (how much power they can store), weight, and charging capabilities.
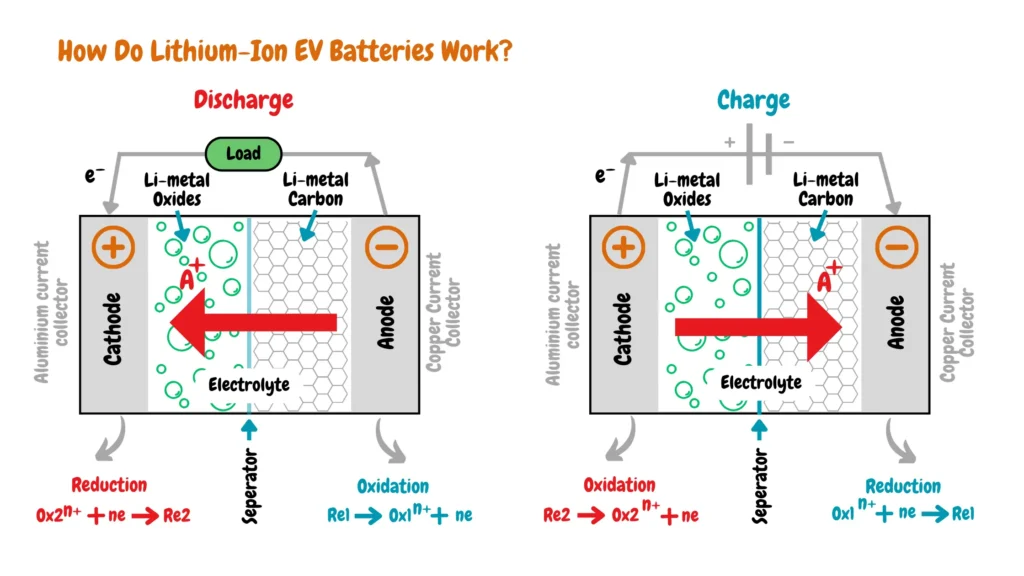
However, they have a limited lifespan and a potential safety risk called “thermal runaway,” where the battery overheats and can catch fire.
There are six types of Lithium-ion batteries, with each type offering distinct advantages and drawbacks.
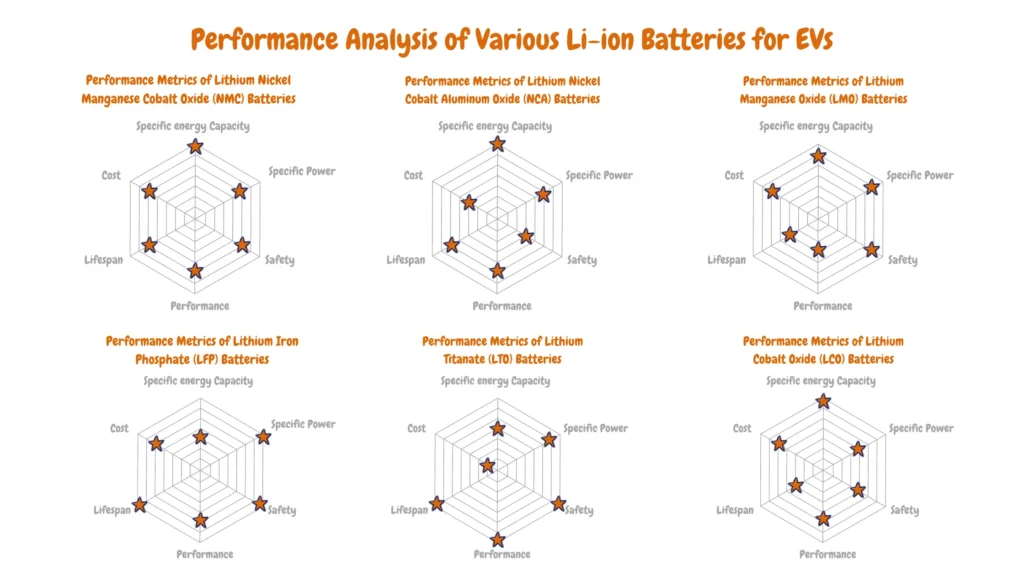
The infographic provides a comparison of the six major lithium-ion cathode technologies: Lithium Nickel Manganese Cobalt Oxide (NMC), Lithium Nickel Cobalt Aluminum Oxide (NCA), Lithium Iron Phosphate (LFP), Lithium Cobalt Oxide (LCO), Lithium Manganese Oxide (LMO), and Lithium Titanate (LTO). Each technology addresses different needs in terms of energy capacity, power, safety, performance, lifespan, cost, and safety.
This dominance of Li-ion technology is evident when looking at major automotive manufacturers. Leading brands like Tesla, Nissan, BMW, Hyundai, and Audi have all heavily relied on Li-ion batteries to power their electric vehicle lineups. This widespread adoption highlights the versatility and effectiveness of Li-ion technology in the current EV market.
Advantages and Disadvantages of Lithium-Ion Batteries
Environmental Impacts of Lithium-Ion Batteries
While lithium-ion batteries power the electric vehicle revolution, their environmental impact can’t be ignored. Concerns primarily revolve around lithium mining, which can deplete local water resources and disrupt ecosystems, and the ethical and environmental issues associated with cobalt mining, particularly in the Democratic Republic of Congo (DRC).
Additionally, Li-ion batteries pose challenges in disposal and recycling due to their hazardous materials, including lithium and heavy metals. Despite ongoing efforts to improve recycling technologies and promote responsible sourcing, the environmental footprint of Li-ion batteries remains a complex issue.
Expert insights emphasize the need for reducing reliance on critical materials like cobalt and developing more efficient recycling methods to ensure a truly sustainable electric vehicle future.
Lithium Iron Phosphate (LiFePO4 or LFP) Batteries
Known for its exceptional safety and longer lifespan compared to other Li-ion chemistries, LFP batteries are becoming increasingly popular. However, they have a lower energy density, meaning they may offer slightly less driving range on a single charge and are slightly heavier.
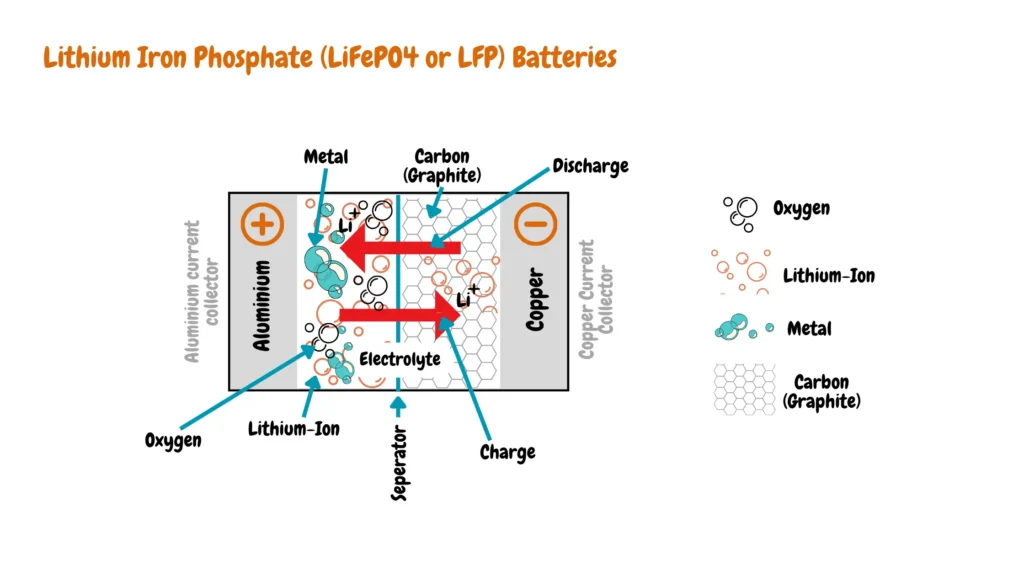
Several leading car manufacturers have already begun incorporating LiFePO4 batteries into their EV lineups. Examples include BYD, China’s Nio, TATA Motors, Hyundai, and even some Tesla models. Some electrical manufacturers such as BMW plan on starting using LFP batteries in 2025. This trend highlights the growing recognition of LiFePO4’s potential to contribute to the development of safer, more cost-effective electric vehicles.
Advantages and Disadvantages of LiFePO4 Battery
Are LiFePO4 Batteries Environmentally Friendly?
LiFePO4 batteries are a strong contender for the title of most environmentally friendly EV battery option. Their key advantage lies in their reduced reliance on cobalt, a material with concerns about mining practices and environmental impact. Beyond just cobalt reduction, LiFePO4 batteries boast a longer lifespan than traditional Lithium-ion batteries. This translates to fewer battery replacements over an EV’s lifetime, reducing the environmental footprint associated with production and disposal. Additionally, their inherent stability simplifies recycling, making them potentially greener at end-of-life.
Lithium Nickel Manganese Cobalt Oxide (NMC) Batteries
Offering a high energy density, NMC batteries are widely used for their good balance of power and range. However, their lifespan is moderate, and some safety concerns exist around potential thermal runaway.
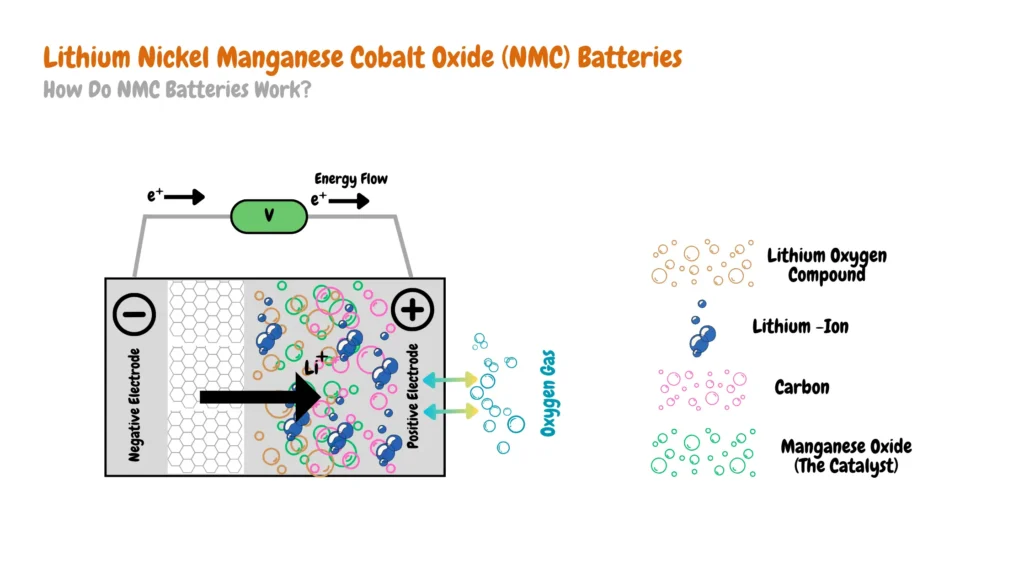
The popularity of NMC technology is evident in several mass-produced electric vehicles. Examples include the Chevrolet Silverado EV, MG ZS EV, Hyundai Kona Electric, and some BMW models. This widespread adoption highlights the effectiveness of NMC batteries in delivering a good balance of range, cost, and thermal management for a variety of EV applications.
Advantages and Disadvantages of NMC Batteries
Is Lithium Nickel and Cobalt Bad for the Environment?
Similar to Li-ion batteries, NMC batteries raise concerns regarding the environmental impact of lithium and cobalt mining. These processes can be energy-intensive and potentially contribute to water and air pollution in some regions.
Lithium Mining: The extraction of lithium can lead to water resource depletion and land degradation.
Cobalt Mining: A significant portion of the world’s cobalt comes from the Democratic Republic of Congo (DRC), where ethical sourcing concerns exist due to potential human rights abuses in some mining operations.
Lithium Manganese Oxide (LMO) Batteries
Prioritizing high power output over energy density, LMO batteries are suitable for applications requiring fast acceleration or bursts of power. However, their overall range may be lower compared to other options.
While not as widely used as Li-ion, NMC, or LiFePO4, LMO batteries have found their place in some early electric vehicle models. Examples include the Mitsubishi i-MiEV and the Kia Soul EV. These applications showcase the potential of LMO batteries for EVs prioritizing fast charging, discharging, and a safe driving experience.
Advantages and Disadvantages of LMO Batteries
Environmental Impacts of Lithium Manganese Oxide (LMO) Batteries
Lithium manganese oxide (LMO) batteries offer reduced cobalt reliance, but concerns persist over their environmental impacts. The extraction of lithium, though less reliant on cobalt, can still be energy-intensive and ecologically disruptive. Additionally, LMO batteries generally exhibit lower energy density compared to NMC batteries, leading to reduced driving ranges in electric vehicles (EVs).
Solid-State Batteries
This emerging technology promises a significant leap in energy density, safety (due to the absence of flammable liquid electrolytes), and lifespan. However, they are currently expensive to produce and not yet commercially viable for large-scale EV adoption.
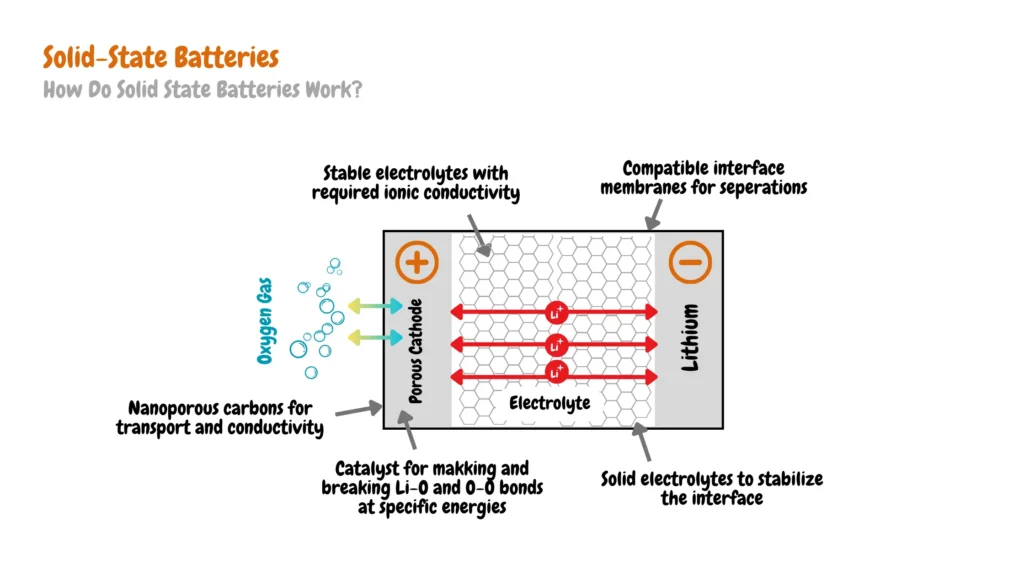
Solid-state batteries have captured the imagination of the electric vehicle (EV) industry for years, holding the promise of a significant leap forward in performance and safety. Often referred to as the “holy grail” for widespread EV adoption, these batteries are actively being pursued by major car manufacturers like Toyota, BMW, Nissan, and Volkswagen, all aiming for commercialization by the end of this decade.
Advantages and Disadvantages of Solid-State Batteries
Sodium-Ion Batteries
Sodium is a more abundant and cheaper resource than lithium. This technology holds promise for potentially higher energy density than current Li-ion batteries. However, sodium-ion batteries are still in the early stages of development, with limited commercial availability.
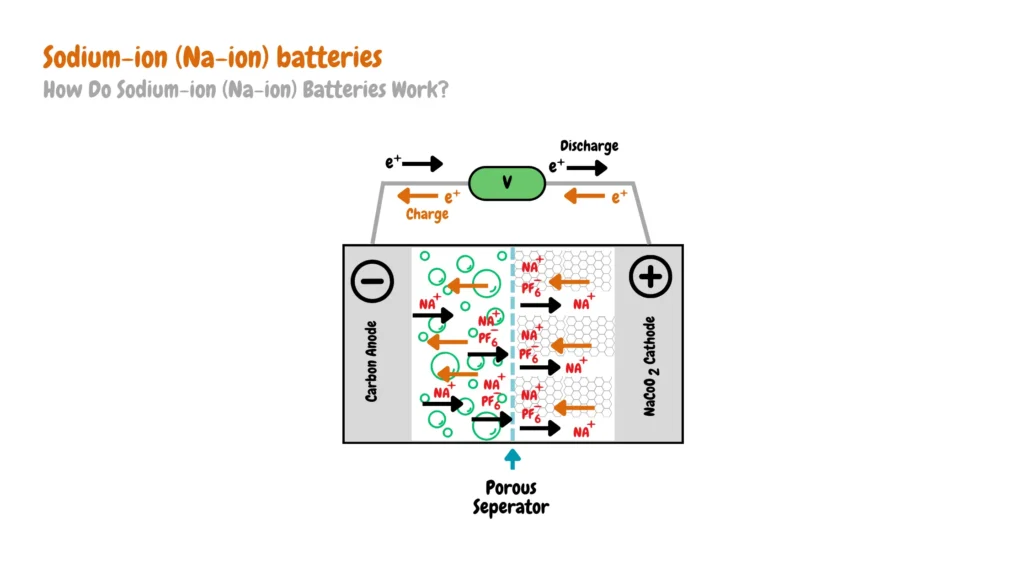
While still in development, NIB technology is already being explored by some manufacturers. Examples include Chinese companies like Jeep, Yiwei, and JMEV. These early applications showcase the potential of NIB batteries, particularly for their environmental benefits. It’s important to note that industry giants like Tesla haven’t publicly announced incorporating NIB technology into their current EV lineup. However, Tesla, along with other major players, is likely closely monitoring advancements in NIB research.
Advantages and Disadvantages of Sodium-Ion Batteries
Comparison of Batteries Used in Electrical Vehicles (Table)
The table below provides a comprehensive comparison of the six electric vehicle battery chemistries, highlighting their key features, strengths, and limitations. The bracketed information emphasizes crucial considerations for each chemistry, including lifespan, safety, and suitability for specific applications.
Electric Vehicle Batteries Features | Lithium-ion (Li-ion) | Lithium Iron Phosphate (LiFePO4 or LFP) | Lithium Nickel Manganese Cobalt Oxide (NMC) | Lithium Manganese Oxide (LMO) | Solid-State Batteries | Sodium-Ion Batteries |
---|---|---|---|---|---|---|
Life Cycle (Cycles) | 1000-2000 (Moderate) | 2000-4000 (longer lifespan) | 1000-2000 (moderate) | 300-700 (shorter lifespan) | Potentially longer than Li-ion (promising research) | Potentially comparable to Li-ion |
Energy Density (Wh/kg) | 100-270 (good balance) | 80-150 (lower than some Li-ion) | 150-220 (higher than standard Li-ion) | 100-150 (similar to standard Li-ion) | Expected to be higher than Li-ion (potentially longer EV range) | Lower than current Li-ion batteries |
Power Density (W/kg) | 250-680 (good power output) | 1000-1800 (excellent) | 250-700 (good) | 1000-4000 (excellent, highest among these) | Expected to be on par with Li-ion | Lower than current Li-ion batteries |
Safety | Potential thermal runaway risk [requires specific handling] | Excellent safety and thermal stability | Safety concerns compared to LFP | Safety concerns compared to LFP | Solid electrolytes eliminate fire risk associated with Li-ion | Similar safety profile to Li-ion batteries |
Charging Efficiency (%) | 80-90 | 90-95 | 80-85 | 80-85 | Not yet applicable (under development) | Not yet applicable (under development) |
Charging Temperature (°C) | 0 to 45 | 0 to 45 | 15 to 45 | 0 to 45 | Not yet applicable (under development) | Not yet applicable (under development) |
Discharging Temperature (°C) | -20 to 60 | -10 to 50 | -20 to 50 | -20 to 50 | Not yet applicable (under development) | Not yet applicable (under development) |
Factors to Consider When Choosing Electric Vehicle Batteries
While you don’t directly choose the battery in an EV (manufacturers select the most suitable option for their vehicle), understanding the key battery considerations can significantly impact your EV buying decision.
Here’s an expert’s insight into what truly matters:
Driving Range
EV battery capacity is pivotal for extended drives. Higher capacities, such as 60 kWh or more, ensure optimal power, enhancing driving range. Nickel Manganese Cobalt (NMC) batteries currently offer the best balance between energy density and affordability, though with trade-offs in lifespan and thermal management requirements.
Charging Time
Faster charging minimizes downtime and maximizes convenience. Lithium-ion (Li-ion) batteries, renowned for their versatility, dominate fast-charging capabilities. However, emerging technologies like Lithium Iron Phosphate (LiFePO4 or LFP) are showcasing comparable rapid-charging abilities, challenging the Li-ion’s dominance.
Battery Chemistry
Different battery chemistries offer unique advantages and disadvantages. Here’s a breakdown of some common types:
- Lithium-ion (Li-ion): (Current Dominating Technology) Li-ion batteries offer a good balance of energy density, weight, and charging capabilities. However, they have a limited lifespan and a potential safety risk called “thermal runaway.”
- Lithium Iron Phosphate (LiFePO4 or LFP): (Prioritizing Safety and Longevity) Known for exceptional safety and longer lifespan, LFP batteries are becoming increasingly popular. They have a lower energy density, translating to a slightly shorter potential range but are a compelling choice for those prioritizing safety and durability.
- Nickel Manganese Cobalt (NMC): (Balancing Range and Cost) This chemistry offers a good balance between energy density and cost, enabling a longer range but with potential trade-offs in lifespan and safety compared to LiFePO4.
Safety Features
Built-in safety measures, including overcharge and short-circuit protection, are critical for driver and vehicle safety. Battery systems with integrated thermal management systems mitigate risks of thermal runaway, ensuring safer operations.
Compatibility and Integration
Seamless integration between EV batteries and vehicle models enhances overall performance and efficiency. Prioritize batteries specifically designed for your EV model to ensure optimal compatibility and performance.
Battery Cost
Consider both upfront costs and long-term ownership expenses, including potential battery replacements. While Li-ion batteries may offer initial affordability, LiFePO4 batteries with extended lifespans may provide better long-term value.
Battery Degradation
Battery robustness is essential for a longer EV lifespan. Opt for batteries with advanced materials and construction to withstand diverse environmental conditions. LiFePO4 batteries, known for safety and longevity, offer reliability over time, reducing the need for frequent replacements.
Environmental Impact
Sustainable battery options with low carbon footprints and recyclable components contribute to a greener future. Sodium-ion batteries show promise for sustainability due to the abundance of sodium compared to lithium, albeit with current performance limitations.
Electric Vehicle Batteries Recycling
The electric vehicle (EV) revolution is driving a hidden but crucial sector: battery recycling. This market, valued at $2.3 billion in 2022, is projected to explode to $9.8 billion by 2028, reflecting a growth rate of 27.3%. This surge is fueled by the rapidly expanding EV market, which will generate a massive wave of retired batteries.
The good news is that we’re equipped to handle this influx. EV batteries boast a remarkable 12-year lifespan and an impressive 99% are collected for responsible processing. The recycling industry itself has a 95% capacity, ensuring most collected batteries are treated. Even more impressive are the material recovery rates: 80% for lithium, a staggering 95% for cobalt, and 95% for nickel.
Several recycling techniques exist, each with its strengths.
- Hydrometallurgical recycling offers the highest recovery rates (90-99%) for key materials and boasts a lower environmental impact. It’s ideal for batteries rich in valuable materials like nickel and cobalt.
- Direct recycling prioritizes environmental friendliness and recovers high-quality materials. While it excels in nickel and cobalt recovery (around 90%), lithium recovery sits at 50%. This method is well-suited for lower-value batteries and unused/aged batteries.
- Pyrometallurgical recycling, the least desirable option, offers the lowest recovery rates and the highest environmental footprint.
The urgency for robust recycling is undeniable. Mineral demand for battery production is expected to increase over thirtyfold by 2040, with lithium demand skyrocketing over fortyfold.
By 2025, an estimated 600,000 metric tons of lithium-ion battery waste from EVs are expected, ballooning to a staggering 11 million metric tons by 2030. However, efficient recycling offers a solution. By 2040, it’s projected to offset 10% of the materials needed for battery production, reducing reliance on virgin materials and their environmental impact. Recovered materials can also be used in battery production, lowering overall battery costs.
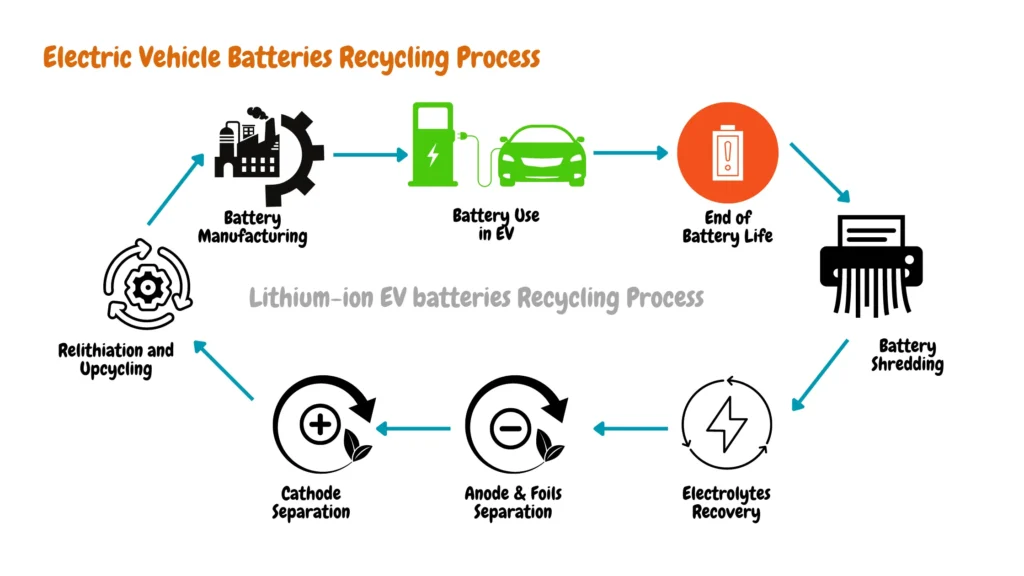
Final Thoughts
The world of electric vehicle batteries is a dynamic landscape, brimming with potential. While Lithium-ion (Li-ion) batteries currently dominate the market, advancements in chemistries like Lithium Iron Phosphate (LiFePO4) for safety and longevity, Nickel Manganese Cobalt (NMC) for extended range, and Lithium Manganese Oxide (LMO) for rapid charging are pushing boundaries. The future holds promise for even more revolutionary options like Solid-State batteries with their potential for faster charging and higher energy density, and Sodium-ion batteries offering a more sustainable alternative.
Car manufacturers are constantly optimizing the electric vehicle equation. While battery chemistry is paramount, factors like cost, energy density, power output, and safety all play a crucial role in selecting the most suitable battery for a specific vehicle. Additionally, design elements like regenerative braking and aerodynamic efficiency significantly impact an EV’s range.
Research and development in electric vehicle batteries are relentlessly focused on key areas:
- Increasing energy density: Packing more power into a smaller space for an extended range.
- Lowering production costs: Making EVs a more accessible and affordable transportation option.
- Enhancing safety standards: Ensuring the highest level of passenger protection.
But the focus extends beyond performance. Sustainable sourcing of raw materials and improved battery recycling practices are critical considerations for a truly environmentally responsible future. As these advancements progress, we can expect a new generation of electric vehicle batteries: longer-range, more affordable, and kinder to the planet.
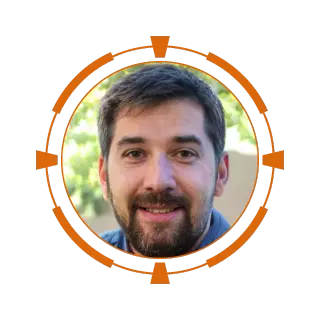
James Ndungu is a certified EV charger installer with over five years of experience in EVSE selection, permitting, and installation. He holds advanced credentials, including certification from the Electric Vehicle Infrastructure Training Program (EVITP) and specialized training in EV charging equipment and installation, as well as diplomas in EV Technology and Engineering Fundamentals of EVs. Since 2021, James has tested dozens of EV chargers and accessories, sharing expert insights into the latest EV charging technologies.