Many leading electric vehicle manufacturers predominantly choose Lithium-Ion Electric Vehicle Batteries, as evidenced by our compilation of the top electric vehicles from 2024 and 2025, along with their respective battery technologies
Electric Vehicle Manufucturer | Country | Electric Vehicle Model | EV Battery Chemistries |
---|---|---|---|
Hyundai | South Korea | 2024 Hyundai Ioniq 6 | Lithium-ion |
BMW | Germany | 2025 BMW i4 | Lithium-ion |
Polestar | Sweden | 2024 Polestar 2 | Lithium-ion |
Tesla | United States | 2024 Tesla Model 3 | Lithium-ion |
Volkswagen | Germany | 2025 Volkswagen ID.7 | Lithium-ion |
Fiat | Italy | 2024 Fiat 500e | Lithium-ion |
Nissan | Japan | 2024 Nissan Leaf | Lithium-ion |
Mini | United Kingdom | 2024 Mini Cooper Electric | Lithium-ion |
Toyota | Japan | 2024 Toyota bZ4X | Lithium-ion |
Table of Contents
How Do Lithium-Ion EV Batteries Work?
Lithium-ion EV batteries operate through reversible chemical reactions between the cathode and anode, facilitating the flow of lithium ions to generate and store electrical energy, thereby powering electric vehicles efficiently and sustainably.
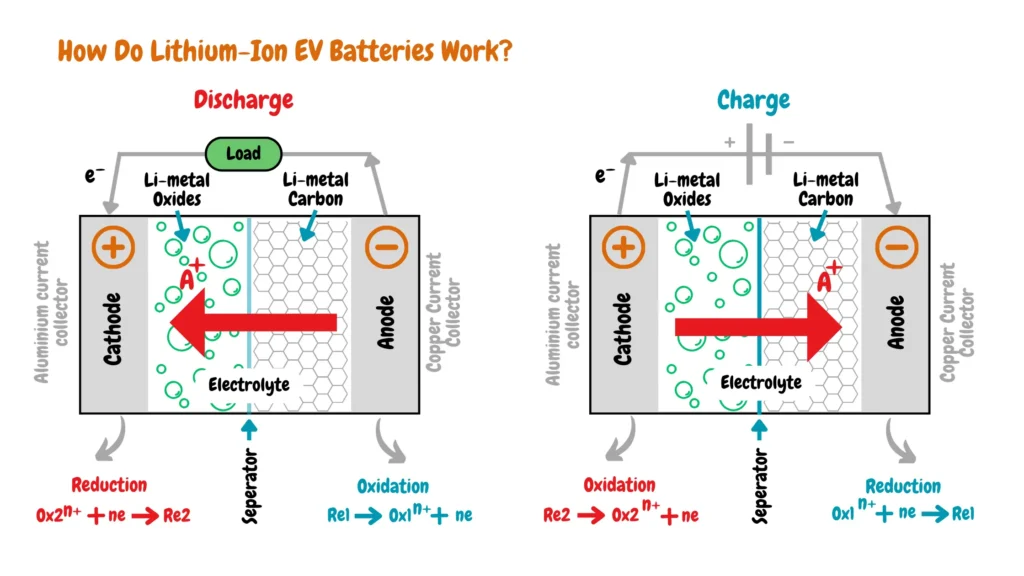
When an EV is turned on, its lithium-ion battery enters the discharging phase, and a chemical reaction occurs within the battery, causing lithium ions to migrate from the cathode to the anode. This flow of lithium ions generates electrical energy that powers the vehicle’s electric motor, propelling it forward.
Conversely, when the EV is turned off or plugged into an electrical outlet for charging, the battery enters the charging phase. In this phase, the chemical reaction reverses, with lithium ions moving from the anode back to the cathode, where they are stored for future use.
Additionally, during regenerative braking, excess kinetic energy produced by the EV is converted into electrical energy. This energy is utilized to recharge the battery, with the flow of lithium ions from the anode to the cathode contributing to energy storage.
Why Is a Lithium-Ion Battery Preferred in Electric Vehicles?
Lithium-ion batteries dominate the electric vehicle (EV) landscape due to their compelling combination of performance and durability. They boast exceptional fast-charging capabilities, a high power-to-weight ratio for optimal performance, and impressive thermal stability that maintains high energy efficiency even under demanding conditions. Additionally, long cycle life and minimal self-discharge solidify their position as the leading EV battery chemistries attractive to leading EV manufacturers.
Another advantage lies in their recyclability, as most components of lithium-ion batteries can be repurposed. However, the industry faces a notable hurdle in the form of material recovery costs, which continue to pose a challenge.
What Type of Lithium-Ion Battery Is Used in EVs?
There are six major types of Lithium-ion batteries used in EVs, they include Lithium Nickel Manganese Cobalt Oxide (NMC), Lithium Iron Phosphate (LFP), Lithium Nickel Cobalt Aluminum Oxide (NCA), Lithium Titanate (LTO), Lithium Cobalt Oxide (LCO), and Lithium Manganese Oxide (LMO).
Lithium-ion batteries are categorized based on the combination of anode and cathode materials as shown in the image below.
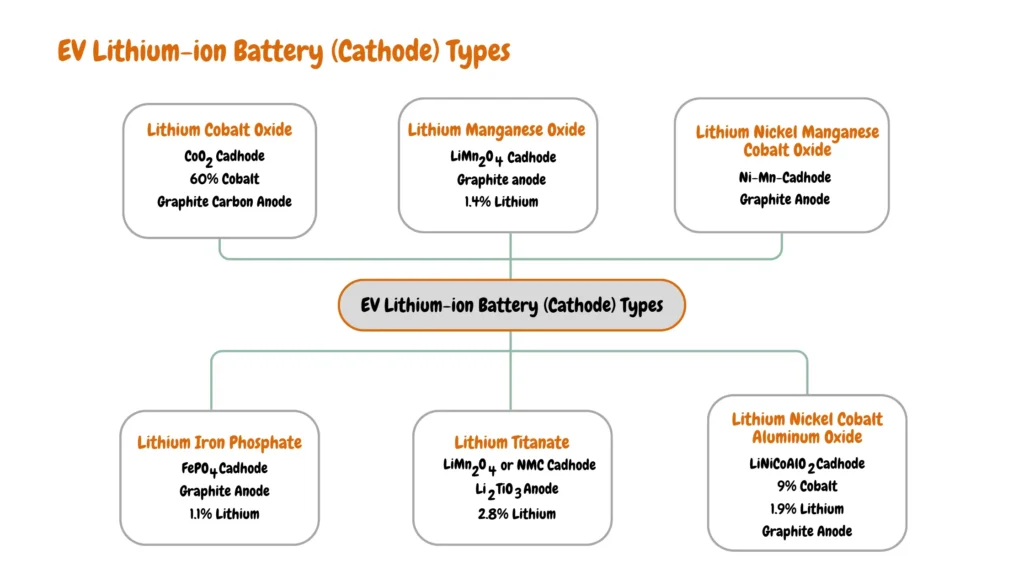
Lithium Nickel Manganese Cobalt Oxide (NMC)
Lithium Nickel Manganese Cobalt Oxide (NMC) batteries are widely preferred in the electric vehicle (EV) industry for their exceptional energy density, translating into extended driving ranges. This chemistry allows EV batteries to store more energy within a compact footprint, enabling vehicles to travel further distances without frequent recharging. However, the inclusion of cobalt in NMC batteries has raised ethical and environmental concerns due to issues surrounding its mining and sustainability.
NMC batteries offer several advantages over other types, notably Lithium Iron Phosphate (LFP) batteries. They boast higher energy density, providing superior range and performance for EVs. Additionally, their smaller size contributes to reduced weight and space requirements within the vehicle. Furthermore, NMC batteries operate at a higher voltage compared to LFP counterparts, resulting in fewer cells needed for equivalent power output, simplifying battery management systems and reducing costs.
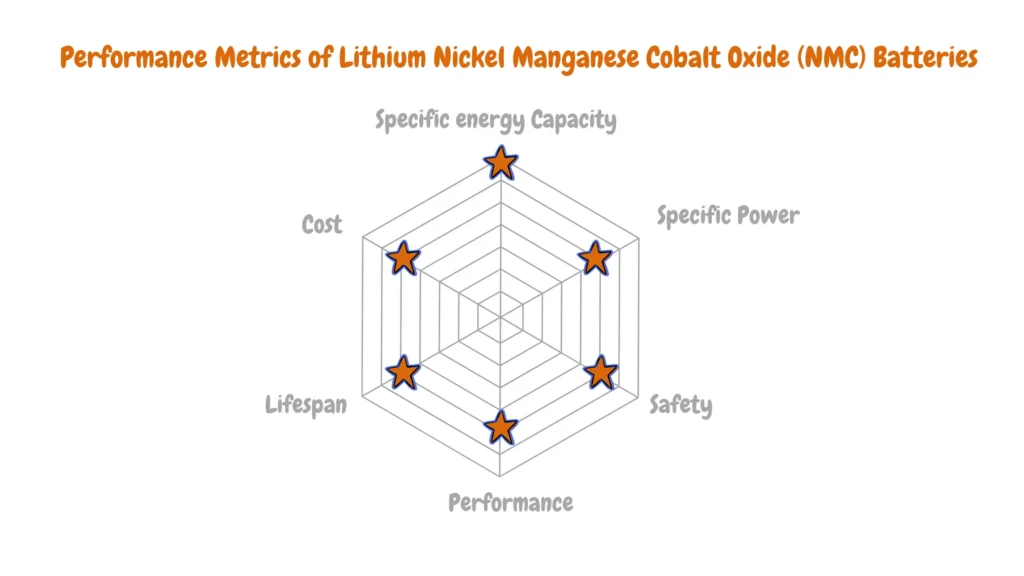
Despite their benefits, NMC batteries exhibit certain drawbacks compared to LFP chemistry. They typically have a shorter lifespan, lasting approximately 3 to 5 years due to faster degradation with each charge-discharge cycle. Moreover, NMC batteries are sensitive to temperature variations, operating optimally within a narrower temperature range of 15°C to 35°C (59°F to 95°F) and are prone to thermal runaway at elevated temperatures. Additionally, the production of NMC batteries involves the use of scarce and costly metals like cobalt and nickel, posing environmental and social sustainability challenges.
Globally, approximately 60% of EVs utilize NMC batteries, highlighting their widespread adoption and importance in advancing electric mobility.
Lithium Iron Phosphate (LFP)
Lithium Iron Phosphate (LFP) batteries are favored in the electric vehicle (EV) domain with electric vehicles such as the Standard Range Tesla Model 3 and Y, MG4, and BYD’s electric cars using lithium Iron Phosphate (LFP) batteries due to their exceptional safety, extended lifespan, and cost-effectiveness.
Lithium Iron Phosphate (LFP) batteries are renowned for their high number of life cycles, reliability, and robustness, making them a preferred choice in various applications. Notably, LFP batteries exhibit greater stability compared to NMC counterparts and boast a wider optimal temperature range of 0°C to 45°C (32°F to 113°F), rendering them suitable for diverse climatic conditions.
In the United States, LFP batteries are particularly advantageous due to their resilience against thermal runaway, a critical concern in regions characterized by high temperatures such as Arizona, Nevada, and Texas. This enhanced safety profile aligns well with climates prone to extreme heat, contributing to their prominence in the market. Moreover, advancements in Battery Management System (BMS) technology and thermal runaway prevention strategies further bolster the safety of LFP batteries, bridging the safety gap vis-à-vis NMC batteries.
Advantages of LFP batteries over NMC include their prolonged lifespan, with the capacity to endure thousands of cycles depending on usage and maintenance. Additionally, their inherently lower risk of fire or explosion, attributed to the absence of cobalt, enhances their safety credentials. Furthermore, LFP batteries are deemed more environmentally friendly as they eschew the use of scarce and costly metals like cobalt and nickel.
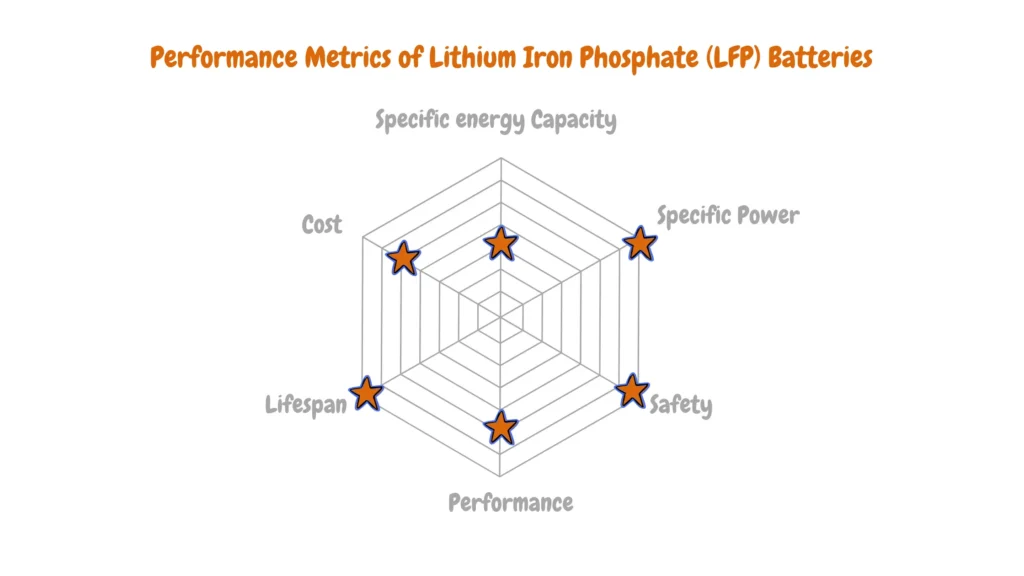
However, LFP batteries exhibit lower energy density compared to NMC counterparts, translating to reduced energy storage capacity within a given space. This results in diminished range and performance for EVs. Additionally, their higher weight relative to NMC batteries contributes to increased vehicle mass, potentially impacting efficiency. Moreover, the lower voltage of LFP batteries necessitates a greater number of cells to achieve equivalent power output, elevating the complexity and cost of battery management systems.
Lithium Nickel Cobalt Aluminum Oxide (NCA)
Lithium Nickel Cobalt Aluminum Oxide (NCA) batteries strike a balance between power and range while potentially containing less cobalt than NMC variants.
These batteries, also referred to as NCA batteries feature a cathode composition comprising nickel, cobalt, and aluminum. Renowned for their longevity, NCA batteries rank among the most energy-dense lithium-ion chemistries, boasting an impressive energy density of up to 260Wh/kg and a nominal voltage of 3.6V. However, their thermal stability is comparatively lower, and their production cost tends to be higher, rendering them less suitable for consumer electronics applications.
Despite this, they stand as a formidable option for Electric Vehicles (EVs), leveraging their energy density. Nevertheless, their integration into EVs necessitates additional safety measures to ensure driver security by monitoring performance and pertinent data.
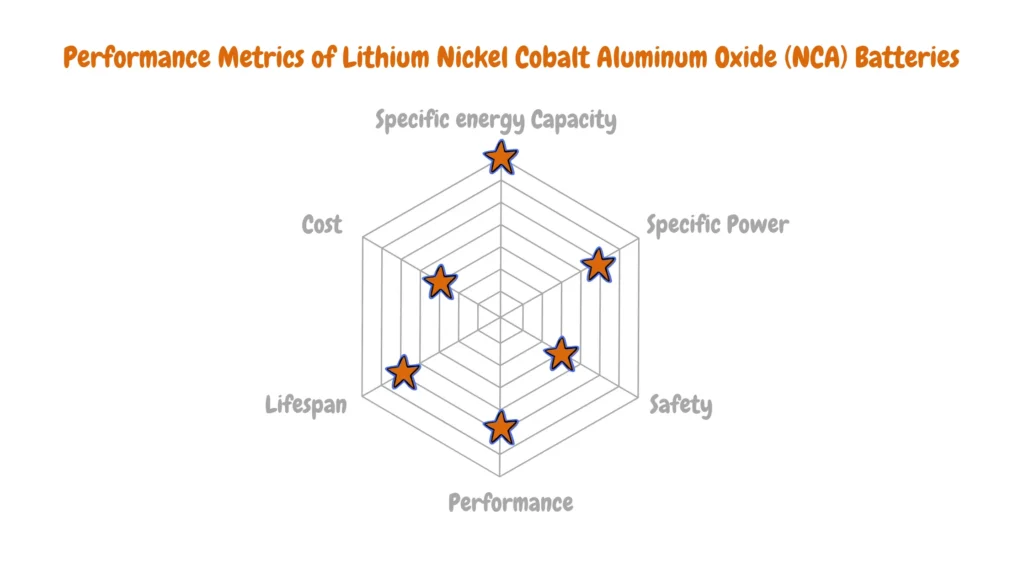
NCA batteries are gaining traction in electric powertrains, notably in vehicles like those manufactured by Tesla, owing to their longevity and energy density. Additionally, they find utility in grid storage applications, capitalizing on their impressive lifespan and energy storage capabilities.
Lithium Cobalt Oxide (LCO)
Lithium Cobalt Oxide (LCO) batteries have long been recognized for their exceptional performance, driven by cobalt as the primary active material in their cathodes. This chemistry, invented in 1991, has garnered widespread usage owing to its impressive energy density, typically ranging between 150-200 Wh/kg.
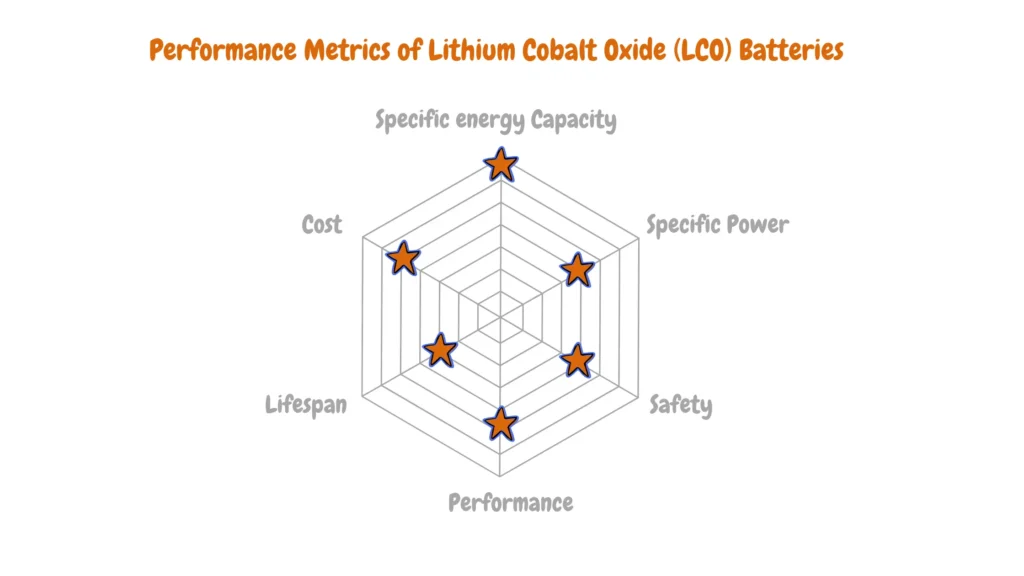
Despite its energy prowess, concerns regarding safety and cobalt supply have emerged, prompting scrutiny and exploration of alternative options. Experts caution against overreliance on LCO batteries, foreseeing a potential cobalt supply shortage due to surging electric vehicle sales, which heavily rely on this chemistry. This anticipated scarcity may precipitate increased costs over time, necessitating a strategic shift towards more sustainable alternatives.
Cobalt’s volatile nature constrains the current handling capabilities of LCO batteries, elevating the risk of overheating. Additionally, LCO batteries exhibit lower thermal stability, rendering them susceptible to higher operating temperatures and overcharging scenarios.
Nevertheless, LCO batteries continue to enjoy high demand in the electric vehicle sector, underscoring their established role in advancing automotive electrification. However, the evolving landscape of battery technologies emphasizes the imperative of diversification and innovation to address sustainability concerns and mitigate supply chain risks associated with cobalt dependency.
Lithium Manganese Oxide (LMO)
Lithium Manganese Oxide (LMO), also referred to as lithium-ion manganese batteries, boasts a reputation for safety and stability. Featuring LiMn2O4 as its cathode material, this chemistry emerged commercially in 1996. Its distinguishing characteristics include low internal resistance and exceptional temperature stability, rendering it safer compared to alternative lithium-ion variants.
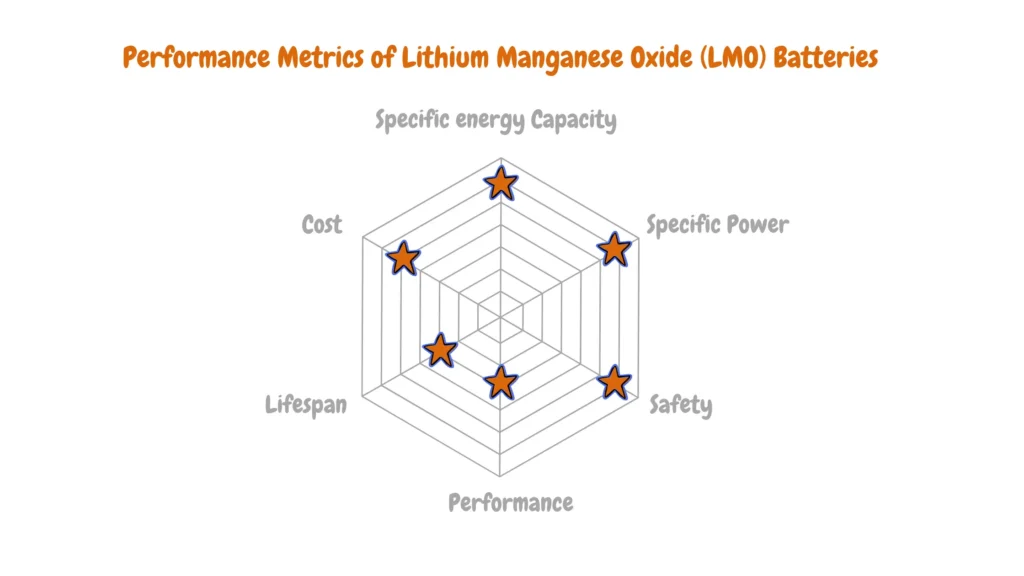
Notably, LMO batteries exhibit a capacity to deliver currents of up to 20-30 Amps, facilitated by their low internal resistance. This attribute enables swift charging and discharging processes, catering to applications requiring rapid energy transfer.
However, LMO batteries are beset by limitations, notably a relatively lower cycle life spanning between 300-700 cycles, coupled with diminished capacity. Consequently, prospects for extensive research and advancement within this battery category remain constrained.
Despite these drawbacks, LMO batteries find widespread utility in scenarios demanding high C-rates, such as power tools. Moreover, they have secured niches in Electric Vehicle (EV) and medical applications, leveraging their unique performance attributes to fulfill specific operational requirements.
Lithium Titanate (LTO)
Lithium Titanate (LTO) represents a cutting-edge technology renowned for its exceptional durability and rapid charging capabilities, although its lower energy density positions it more favorably for specific applications such as long-distance electric vehicles.
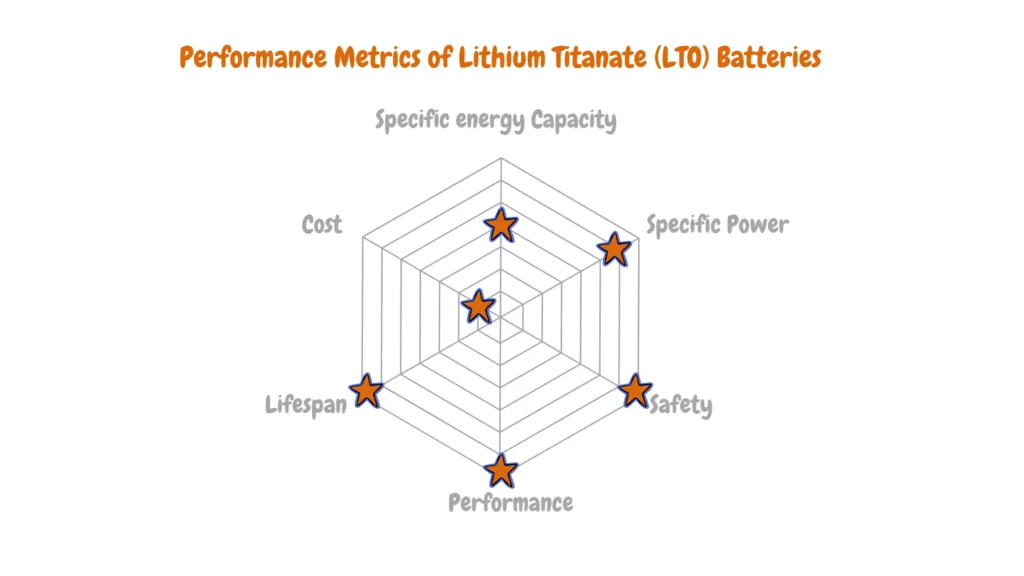
This innovative lithium-ion chemistry, also known as li-titanate, incorporates advanced nanotechnology, replacing conventional graphite in the anode with lithium titanate as the active material. The unique structure of lithium titanate affords a significantly larger surface area, facilitating the swift ingress and egress of electrons. Consequently, LTO batteries can undergo rapid charging and discharging cycles without compromising safety, setting a benchmark for high-performance energy storage solutions.
Despite its impressive attributes, LTO batteries face limitations concerning nominal voltage and energy density, rated at 2.4V and 50-80Wh/kg, respectively. Nonetheless, their standout feature lies in their capability to deliver discharge rates exceeding 30 C for short durations. Moreover, the integration of advanced nanotechnology renders LTO batteries among the safest lithium-ion chemistries available today.
Major players in the electric vehicle manufacturing sector, such as Mitsubishi and Honda, have embraced li-titanate batteries, underscoring their potential within the transportation industry, particularly for electric buses in public transit networks.
Comparisons of Different Types of Li-ion Batteries Used in EVs
The table below offers a detailed comparison of lithium-ion battery chemistries used in EVs across key performance metrics: These metrics include energy density (Wh/kg), power density (W/kg), cycle life (number of charge/discharge cycles), C-rates (charge and discharge rates), thermal stability, recyclability, capacity retention, and cost.
Lithium Iron Phosphate (LFP) | Lithium Nickel Manganese Cobalt Oxide (NMC) | Lithium Cobalt Oxide (LCO) | Lithium Titanate Oxide (LTO) | Lithium Nickel Cobalt Aluminum Oxide (NCA) | |
---|---|---|---|---|---|
Energy Density | 90-150 Wh/kg | 150-220 Wh/kg | 150-250 Wh/kg | 50-100 Wh/kg | 200-280 Wh/kg |
Power Density | 300-500 Wh/kg | 600-1000 Wh/kg | 300-500 Wh/kg | 1000-3000 Wh/kg | 800-1500 Wh/kg |
Cycle Life | 2000-4000 | 1000-2000 | 500-1000 | 5000-10000 | 1000-2000 |
Charge and Discharge Rates | 1C-3C | 1C-3C | 1C-3C | 5C-50C | 1C-3C |
Thermal Stability | High | Medium | Low | Very High | High |
Recyclability | Yes | Yes | Yes | Yes | Yes |
Capacity Retention | Low | Medium | Varies | Lowest | Medium |
Cost | $100-150/kWh | $150-200/kWh | $200-250/kWh | $300-400/kWh | $200-250/kWh |
Which Lithium-Ion Battery Is Best for Electric Vehicles?
There isn’t a single “best” lithium-ion battery for electric vehicles (EVs) because each chemistry offers advantages and disadvantages depending on the specific needs of the vehicle.
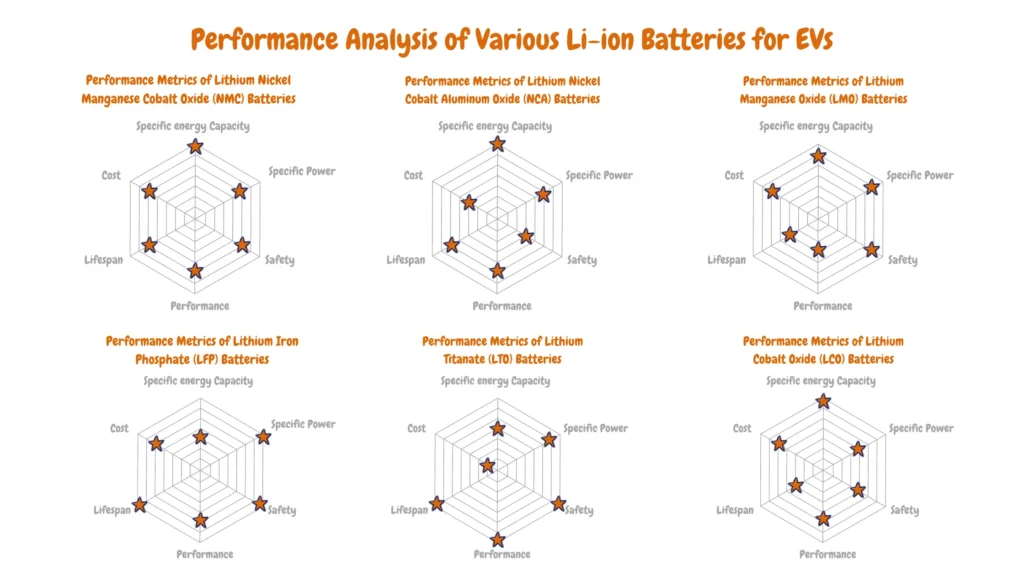
As you can see from the Performance Analysis of Various Li-ion Batteries for EVs radar charts above, there are diverse key metrics like performance, energy density (range), power density (acceleration), safety, cost, and cycle life (lifespan) with each lithium-ion battery having an advantage over the other. Electric vehicle manufacturers analyze this data to determine the most suitable lithium-ion battery type for a particular EV depending on the electric vehicle vehicle design goals, target market, and cost.
Best Lithium-Ion Batteries for Long-Distance Electric Vehicles
Lithium Nickel Manganese Cobalt Oxide (NMC) batteries are the dominant choice for EVs seeking maximized energy density (Wh/kg), translating to extended driving range. However, concerns regarding cobalt content and thermal stability are considerations for manufacturers.
Safest and Most Affordable Lithium-Ion Batteries for Electric Cars
Lithium Iron Phosphate (LFP) batteries offer a compelling alternative due to their inherent thermal stability and extended cycle life. This translates to exceptional safety characteristics and lower long-term battery replacement costs.
Environmental Friendly Lithium-Ion EV Batteries
Lithium Nickel Cobalt Aluminum Oxide (NCA) batteries present a potential compromise. They offer a respectable energy density for a reasonable driving range while boasting improved power density (W/kg) compared to LFP. Additionally, they typically contain lower cobalt content compared to NMC, addressing some ethical and environmental concerns.
How Long Do EV Lithium-Ion Batteries Last?
The lifespan of lithium-ion EV batteries makes them ideal for electric vehicles, with an average durability ranging between 10 to 20 years under proper usage and care.
Similar to lithium-ion batteries in electronic devices, it’s advisable to avoid extreme charging cycles, maintaining the battery’s state of charge (SOC) between 20 to 80%. Frequent high-voltage charging and deep discharges can accelerate battery degradation, as can exposure to extreme temperatures.
Moreover, driving style significantly influences battery longevity. Factors such as frequent use of heating or cooling systems can impact the battery’s range and overall health.
To prolong battery life, drivers should adopt practices like balanced charging, temperature control, and smooth acceleration. Additionally, most manufacturers provide warranties for their batteries, typically spanning 5–8 years, offering added assurance to EV owners.
Frequently Asked Questions.
What are the components of a lithium-ion (Li-ion) cell?
Li-ion cells consist of four main components: Cathode, Anode, Electrolyte, and Separator. These components work together to facilitate the flow of current within the battery.
What is the role of the cathode in a Li-ion cell?
The cathode plays a crucial role in determining the capacity and voltage of the Li-ion battery. It houses the active material responsible for the chemical reactions that enable current flow. The cathode’s capacity depends on the size of the active material, while its voltage is influenced by the cathode’s elemental composition.
What is the anode and its function in a Li-ion cell?
The anode, similar to the cathode, is coated with an active material and serves as the negative electrode. It determines the rate of charge and discharge of the battery. Maximizing the surface area of the anode enhances the flow of lithium ions between the cathode and anode, impacting the battery’s performance.
What role does the electrolyte play in a Li-ion cell?
The electrolyte solution, typically a lithium salt dissolved in an organic solvent, facilitates the movement of ions between the cathode and anode. During charging, lithium ions migrate from the cathode to the anode through the electrolyte, while the reverse occurs during discharge. This movement of ions enables the flow of electric current within the battery.
What is the function of the separator in a Li-ion cell?
The separator physically isolates the cathode and anode while allowing the passage of ions between them. This prevents electrical contact between the electrodes while ensuring the flow of current. In case of overheating, the separator may act as a safety feature, halting the flow of current and safeguarding the battery from potential fire hazards.
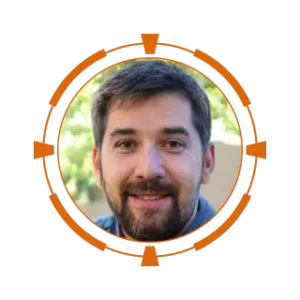
About the Author: James Ndungu
James Ndungu, founder and editor-in-chief of Electric Vehicle Geek, brings over five years of hands-on experience in Electric Vehicle Supply Equipment (EVSE) selection, permitting, and installation. He specializes in assisting businesses and homeowners in the United States with a seamless transition to electric vehicles.
As a certified EV charger installer and holder of advanced certifications, including the EVITP (Electric Vehicle Infrastructure Training Program), Diploma in Electric Vehicle Technology, and Diploma in Engineering Fundamentals of Electric Vehicles, I provide expert guidance and in-depth reviews on the latest EV charging equipment.