Here’s how we set out to plan, design, and install a solar-powered EV charging system for our Level 2 EV charger, to power our electric vehicle and reduce reliance on the grid.
We wanted to build a future-proof bi-directional EV charging solar-powered EV charging system that was both practical and sustainable, integrating a 10kW rooftop solar array with our home’s single-phase electrical grid to support Level 2 solar-powered EV charging. And now, we’re excited to share every detail of how we did it.
We’ll cover the whole process that we have to do to install a solar-powered EV charging system, from assessing our rooftop’s solar potential to calculating our system’s energy output, with plenty of practical tips along the way. You’ll also better understand the technical aspects, like shadow analysis, array sizing, and electrical considerations.
We will also introduce to you free software that makes our work easier when it comes to site analysis, and array design, even if you’re new to solar energy, you’ll be able to follow along.
Table of Contents
- Best EV Car Chargers for Solar Integration
- Our Solar-Powered EV Charging System Feasibility Study
- Technical Viability of Our Solar-Powered EV Charging System
- Recommendations from our Feasibility Report
- Solar Capacity for Level 2 EV Charging
- Our Solar EV Charging Station System Design
- Solar-Powered EV Charging System Equipment List
- Our Solar-Powered EV Charging System Circuit Diagram
- Our Solar-Powered EV Charging System Energy Yield
- Financial Analysis of Our Solar-Powered EV Charging System Project
- Financial Incentives, Rebates, and Tax Credits for Solar Installations
Best EV Car Chargers for Solar Integration
Are you looking for the best EV chargers for solar integration, here is a list of the best chargers we recommend for solar-powered homes, these chargers are designed for seamless solar integration, offering efficient and eco-friendly charging. Some models such as Tesla Chargers and Wallbox Chargers include advanced monitoring systems, allowing you to track energy usage and optimize charging for maximum efficiency and cost savings.
Tesla Universal Wall Connector Review
Tesla Gen 3 Wall Connector Review
Wallbox Pulsar Plus 40-Amp EV Charger Review
Emporia EV Charger Review
Emporia Level 2 Charger with NACS Review
JuiceBox 40 Amp EV Charger Review
Wallbox Pulsar Plus 48-Amp EV Charger Review
JuiceBox 32 Amp EV Charger Review
Enphase IQ 50 EV Charger Review
Our Solar-Powered EV Charging System Feasibility Study
Before installation and purchase of solar power EV charging equipment, we first conducted a feasibility study to assess the practicability and benefits of installation of a solar-powered EV charging system on our property.
Technical Viability of Our Solar-Powered EV Charging System
We conducted an in-depth technical viability assessment of our solar-powered EV charging system to ensure optimal design and functionality. The evaluation encompassed the following key steps:
Property Analysis
We began by evaluating the available space on our property for solar panel installation. Using Google Earth, we examined both the roof and ground areas, considering factors such as size, orientation, shading, and elevation. Additionally, we utilized Google Earth’s measurement tools to calculate the total area of our rooftop, as shown in the image below:
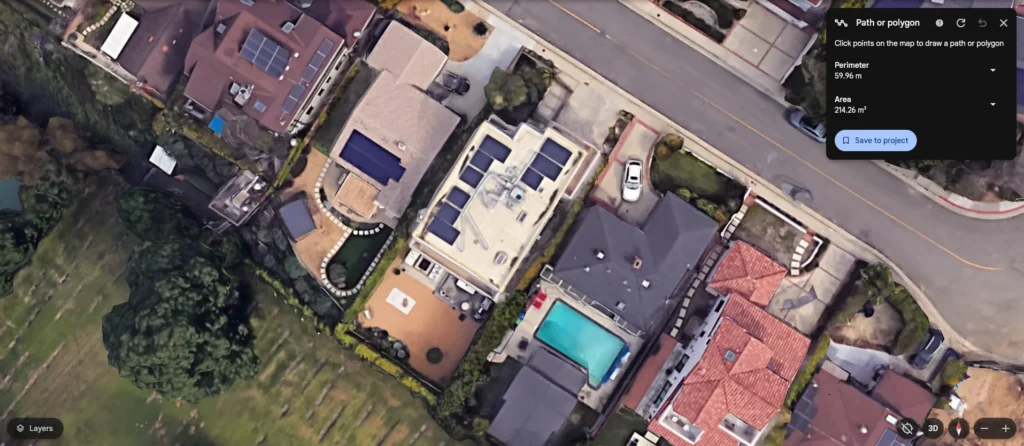
As shown in the image above, the roof’s clearance, spacing (214.26m2), and elevation are key factors in our decision. The roof’s elevation provides a distinct advantage by positioning the panels above potential obstructions, such as trees or neighboring buildings, ensuring optimal sunlight exposure. While we did assess the ground area, challenges such as traffic, slope, and other obstructions made it less ideal for installation.
Ultimately, we opted for the rooftop due to its flat surface and favorable elevation, offering ample space for panel installation without requiring additional structural adjustments. In comparison to ground-mounted systems, rooftop installations avoid issues like ground clearing and obstructions from nearby structures or vegetation, while also enabling a more efficient connection to the electrical system.
Roof Assessment
We then conducted a manual roof assessment to check its suitability for solar panel installation. Our manual roof assessment also included evaluating obstacles in the roof and the roof’s orientation to ensure maximum solar exposure throughout the day by considering its direction and angle relative to the sun. Additionally, we examined the roof’s material and structural integrity. A strong, weather-resistant roof is essential for supporting the panels, and proper orientation ensures optimal energy generation.
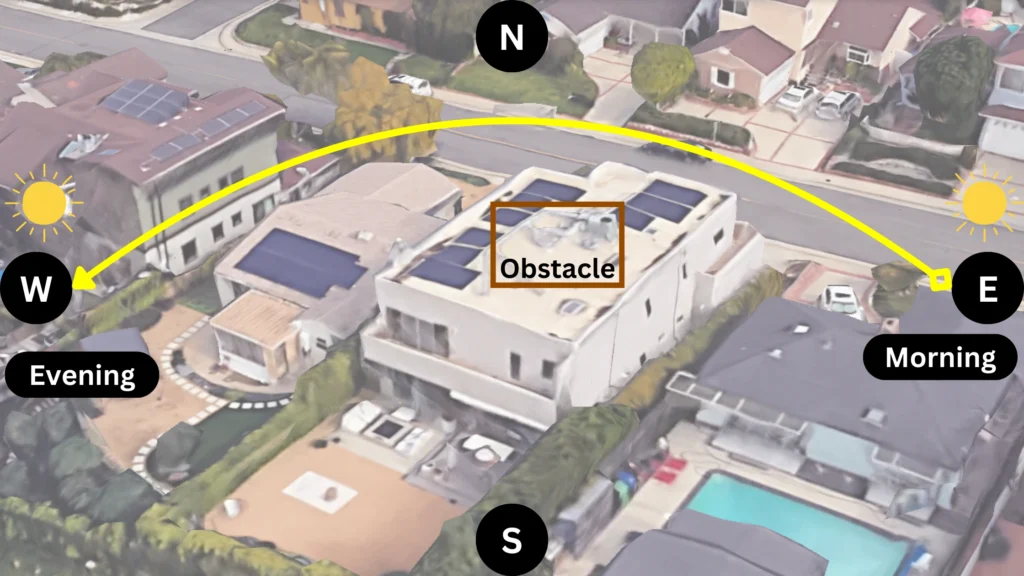
As seen in the annotated roof orientation image above, we identified an obstacle in the center that prevents us from installing panels there. The image also highlights the roof’s east, west, north, and south orientations, with arrows indicating the sun’s path from east to west. Due to these constraints, we chose to install panels on the east side to capture the morning sun and on the west side to capture the evening sun. This strategic placement ensures optimal solar energy capture and improves the overall efficiency of our solar system.
Sun Path Analysis
We also generated a sun path chart to track the sun’s trajectory throughout the day and across seasons shown below.
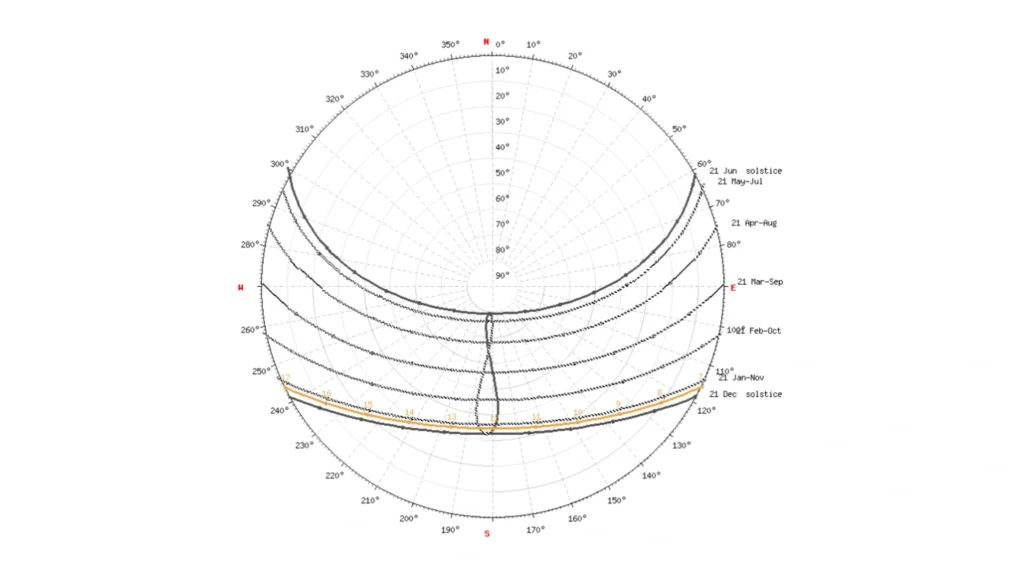
This chart helped us determine the best times for solar panel exposure, allowing us to optimize placement for maximum energy production. By comparing this data with our roof’s orientation and obstacles, we made informed decisions on solar placement on our roof to enhance efficiency.
Solar Radiation Analysis
An important study we also conducted was the solar radiation analysis of our property to determine its solar exposure throughout the year. This allowed for accurate predictions of energy production. As shown in the feasibility study table below, our property (solar installation site) receives an average solar radiation of approximately 5.97 kWh/m²/day over the year.
Month | Solar Radiation (kWh/m2/day) |
---|---|
Jan | 3.85 k/m/d |
Feb | 4.31 k/m/d |
March | 6.13 k/m/d |
April | 7.29 k/m/d |
May | 7.6 k/m/d |
June | 7.41 k/m/d |
July | 7.09 k/m/d |
Aug | 6.55 k/m/d |
Sep | 6.08 k/m/d |
Oct | 5.29 k/m/d |
Nov | 4.22 k/m/d |
Dec | 3.85 k/m/d |
Average | 5.97 kWh/m²/day |
From the figures above, we also created a solar radiation bar chart shown below to help us easily visualize the monthly solar radiation. This chart allows for a clear comparison of solar exposure throughout the year, highlighting seasonal variations.
In our case, the months of May (7.6 kWh/m²/day) and June (7.41 kWh/m²/day) stand out as the peak periods of solar radiation, offering the highest potential for energy generation. On the other hand, December (3.85 kWh/m²/day) and January (3.85 kWh/m²/day) experience the lowest radiation levels, which may require adjustments in energy planning to accommodate these less sunny months.
By visualizing the data, we can optimize the solar system’s performance, ensuring a reliable energy supply for EV charging even during the seasonal dips in solar exposure.
Electrical Panel Assessment for Solar Integration
We conducted a thorough feasibility study of the main electrical panel to assess its capacity, condition, and overall suitability for integrating a solar power system. This evaluation provided an in-depth understanding of the panel’s current state and highlighted necessary upgrades or the potential need for a sub-panel to support the main electrical panel. The goal was to ensure a safe, efficient, and compliant integration of the solar power system.
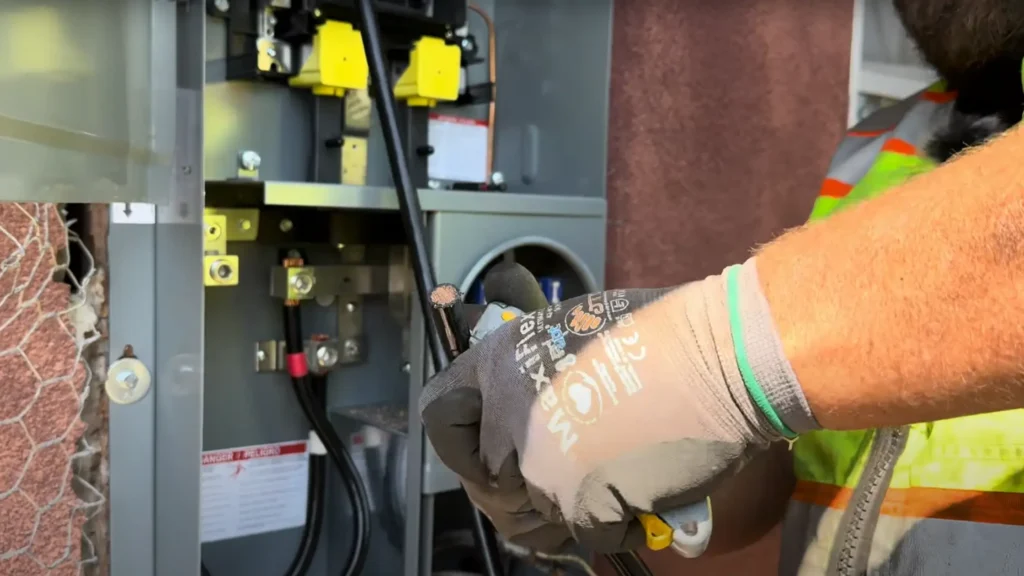
The study focused on the following critical considerations:
Incomer Cable Capacity (Single Phase 240V)
We evaluated the capacity of the single-phase 240V incomer cable to ensure it could handle the combined load from the utility grid and the solar power system. The cable’s cross-sectional area and ampacity were analyzed to determine if upgrades were needed to support the increased current flow.
Connection of Incomer Cable to Electrical Meter
The connection point of the incomer cable to the electrical meter was inspected for integrity and compliance with local electrical codes. This included checking for proper grounding, secure connections, and any signs of wear or damage that might affect the system’s performance or safety.
Surge Protection Devices (SPDs)
We assessed the need for surge protection devices to safeguard the system from voltage spikes originating from the utility grid. Properly rated SPDs were evaluated for installation at key points, such as the main panel and near-sensitive equipment, to protect both the solar system and household appliances.
Cable Routing and Cable Management
The routing of cables from the solar inverter, incomer cable, and other connections was planned to ensure a neat and efficient layout. Attention was given to minimizing cable lengths, avoiding sharp bends, and securing cables to reduce wear and maintain safety. Appropriate conduits and cable trays were identified to manage cables effectively and protect them from environmental damage.
Switch Slots
The availability of switch slots in the electrical panel was checked to confirm space for the solar back feed breaker and any additional breakers needed for future expansions, such as EV chargers or other renewable energy systems.
Electrical Connection to the House
We reviewed the electrical connection between the panel and the house to verify compatibility with the solar system. This included ensuring the internal wiring could handle the combined load, inspecting grounding and bonding systems, and confirming that the existing setup complied with safety standards.
Utility Data Collection and Power Demand Calculation
To assess the property’s energy needs, we analyzed 12 months of utility bills and determined that the average energy consumption is 600 kWh per month. This figure reflects the energy demands of our 2000 sqft home featuring two kitchens, two washers/dryers, a media cabinet, three computers, a surveillance system, two TVs, two mini splits, and a range of smart home features for lighting, audio, and automation. Energy usage is partially offset by a gas stove and gas heater.
EV Charger Power Demands.
Adding a Level 2 EV charger to the home introduces additional energy requirements. Our Level 2 charger of choice operates at up to 40 amps on a 240V circuit, delivering a charge rate of 9.6 kW per hour. To ensure sufficient capacity for seamless integration, we calculated the total energy demand, factoring in the EV charging load.
Additional Energy Consumption for EV Charging
Here is how we calculated our EV charger power demands:
Step 1: Energy Consumption Per Charging Session
A single charging session from 10% to 80% battery capacity takes approximately 4.5 hours. With the Level 2 charger delivering 9.6 kW per hour, the energy consumed per session is:
9.6kW × 4.5hours = 43.2kWh/session.
Step 2: Monthly Energy Consumption
Assuming 15 charging sessions per month (with a Model 3 driving approximately 1,000 miles per month and a Model Y driving about 1,500 miles per month), the total energy consumption for charging would be:
43.2kWh/session × 15sessions/month = 648kWh/month.
Total Energy Demand
Combining the household’s baseline consumption of 600 kWh per month with the EV charging load of 648 kWh monthly, the total energy demand rises to 1,248 kWh monthly.
Recommendations from our Feasibility Report
Based on our analysis, the household’s baseline energy consumption of 600 kWh per month, combined with the EV charging load of 648 kWh per month, results in a total monthly energy demand of 1,248 kWh. This translates to an average daily consumption of 41 kWh (1,248 kWh ÷ 30 days).
A 10 kW solar system is recommended for this project, as it can produce approximately 40–44 kWh per day, depending on sunlight conditions and system efficiency. This capacity is sufficient to meet the household’s energy requirements, including EV charging.
Key Supporting Factors
- Technical Feasibility:
- The property meets all technical requirements for installing a 10kW solar-powered system.
- The roof structure is suitable for panel installation with a little designs adjustment for solar panel installation, the roof offers sufficient exposure to sunlight as confirmed by the sun path and solar radiation analysis.
- The electrical panel, incomer cable capacity, and surge protection devices are adequate or can be upgraded to ensure safe integration with the existing infrastructure.
- Energy Efficiency and Power Demands:
- Utility data collection and power demand calculations confirm that the system can reliably meet EV charger power requirements while reducing reliance on grid electricity.
- Cable Management and Connection:
- Detailed assessments of cable routing, connection slots, and electrical integration confirm that the system can be seamlessly incorporated into the property’s existing setup.
Recommendations for Implementation
To optimize performance and reliability, we further recommend:
- Installing a solar production and usage meter with advanced monitoring features to track solar energy production, usage patterns, and system performance.
- Evaluating opportunities for financial incentives or tax credits to reduce upfront costs.
- Ensuring the electrical panel and cabling are upgraded as needed to handle the increased energy load.
This approach ensures sustainability, efficiency, and cost-effectiveness while fully supporting the household’s energy needs.
Solar Capacity for Level 2 EV Charging
We opted for a 10kW solar system for our Level 2 EV charger because it meets our EV charger’s power needs and provides enough energy to charge the EV during the day while also powering the home as shown in our feasibility study report above. This setup ensures optimal energy yield, especially on sunny days, and can handle backup needs during cloudy periods.
How Many Solar Panels Do You Need for a Solar-Powered EV Charging System?
When designing a solar-powered system for our Level 2 EV charger, one key consideration is how many solar panels we’ll need to generate the required energy. For a 10 kW system, the number of panels will depend on the wattage of the solar panels.
Here’s how to calculate it:
Number of Panels = System Size (kW) / Panel Rating (kW)
For instance, if we select panels with a rating of 350 watts (0.35 kW), we would need approximately 29 panels to meet the 10 kW system requirement.
Number of Panels = 10kW/ 0.35W =28.57 Panels ≈ 29 Panels
To give you a better idea, we’ve created a table with the number of panels needed based on different panel ratings:
Panel Rating | Number of Panels Needed |
---|---|
300W | 34 Panels |
325W | 31 Panels |
350W | 29 Panels |
400W | 25 Panels |
Our Solar EV Charging Station System Design
Our solar EV charging station design was predominantly shaped by the configuration of our solar panel connections. We opted for a series-parallel configuration to optimize energy efficiency. Given that we were utilizing a Canadian Solar inverter with 3 MPPTs (Maximum Power Point Trackers) and 3 strings, we strategically increased the capacity and voltage of the system to ensure alignment with the current and voltage requirements.
Our Solar EV Charging Station System Solar Strings
With each solar panel rated at 325W, we needed to allocate panels per string in a way that would balance both current and voltage. The calculation (31/3 = 10.33) suggested that each string should consist of 11 panels, ensuring optimal performance. Therefore, the total number of panels for our solar EV charging station system was adjusted to reflect this design, resulting in a final count greater than the initially expected 33 panels.
The Image below shows our Solar EV charging station system solar strings design and connection:
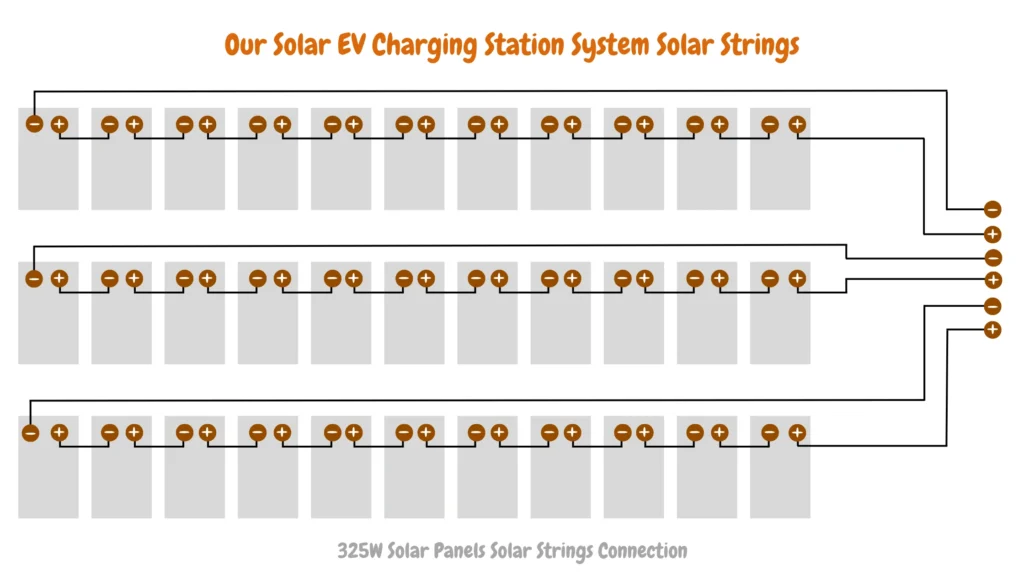
Our Solar EV Charging Station Solar Placement (Roof)
To ensure optimal performance, we carefully considered the placement of our solar panels for the EV charging station. Our primary goal was to maximize sun exposure, which was influenced by our roof’s unique features. Based on the feasibility report, we found that the roof had a central obstacle that made it impractical to place all the solar panels on either the eastern or western side.
To solve this based on our shadow analysis of the obstacle, we strategically placed the first and second strings of solar panels on the western side to capture the afternoon sunlight, while the third string was positioned on the eastern side to maximize morning sun exposure. This layout optimizes the overall energy production throughout the day.
Additionally, we took into account the necessary tilt angle (or inclination) for the solar panels. The design of the panel arrays on both the eastern and western sides was essential for ensuring optimal energy capture. While a high mounting structure was a potential option, we opted for a low-profile mounting system due to the high winds in our area, which could make a raised structure unstable.
For the mounting system, we chose a fixed, roof-mounted structure made of galvanized steel. Given the prevalence of strong winds and the increasing frequency of hurricanes, we prioritized stability and durability over a more vulnerable mounting solution.
The image below illustrates the strategic placement of our solar panel arrays on the roof of our home:
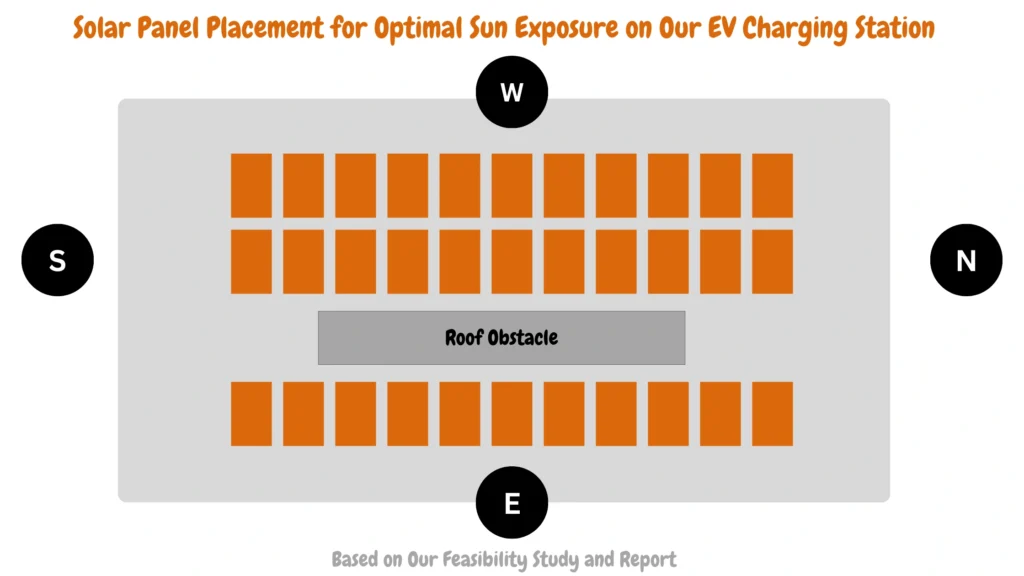
Solar-Powered EV Charging System Equipment List
The installation of our solar-powered Level 2 EV charging system was completed in 2025, following a year of meticulous planning. During this period, we had partially acquired the necessary materials for the system. Reflecting on the process, it became evident that a comprehensive and organized materials list would have significantly optimized our workflow.
To assist future DIY enthusiasts and professional solar panel installers in executing similar projects efficiently, we have compiled a detailed table of materials required for a solar-powered Level 2 EV charging system.
List of Materials for a 10kW Solar-Powered EV Charging System | Quantity |
---|---|
Solar Panels (325W) | 33 |
Roof-mounted racks or ground-mounted system | 33 |
Grid-tied inverter with MPPT (Maximum Power Point Tracking) technology | 1 |
DC Distribution Board | 1 |
AC Distribution Board | 1 |
6 AWG Copper XLPE Solar Cable | 50M |
3-Core 25mm² Armoured AC Power Cable | 50M |
Grounding System Kit (Earthing System Kit, Earthing Cable, and Earthing Strip) | 1 |
Safety Components (Surge Protection Device, Miniature Circuit Breaker, DC Fuses, DC and AC Disconnect Switches, MC4 Connectors) | 1 |
Lightning Arrestor | 1 |
Optional Solar-Powered EV Charging System Equipment List (Upgrade)
Charge Controller | 1 |
Battery Storage | 1 |
Bi-directional Meter | 1 |
Solar Monitoring System | 1 |
Best EV Car Chargers For Solar Integration
Best chargers for solar integration These are the units we’d recommend
Our Solar-Powered EV Charging System Circuit Diagram
Our solar-powered EV charging system circuit diagram shown below is designed to integrate solar energy generation with the electrical grid and provide a sustainable solution for our home EV charging.
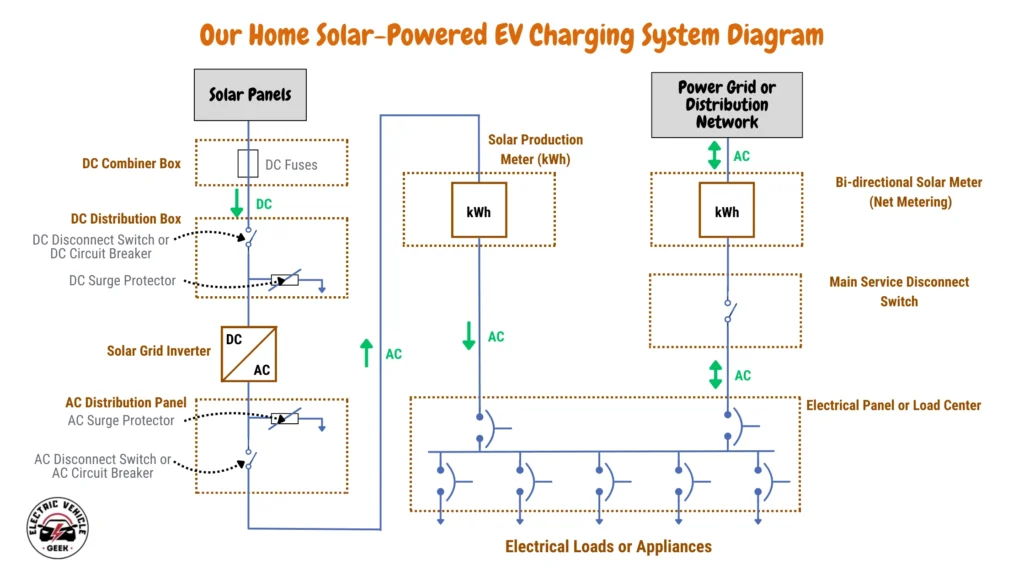
Here’s a breakdown of the system and how it works seamlessly to power our electric vehicle while ensuring safety, efficiency, and grid interaction.
Solar Energy Generation
The process begins with solar panels, located at the top left of the diagram. These panels capture sunlight and convert it into direct current (DC) electricity. The energy produced is then routed through several key components for protection and distribution.
- DC Combiner Box: After being generated by the panels, the DC electricity flows through fuses for safety before entering the DC Combiner Box. This box consolidates the DC output from multiple strings of solar panels into one output.
- DC Distribution Box: The combined DC power is then directed to the DC Distribution Box, which contains crucial components for system protection:
- DC Disconnect Switch: This switch allows for manual disconnection of the DC power, serving as an on/off switch.
- DC Surge Protector: Protects the system from voltage spikes caused by lightning or other electrical issues.
Power Conversion and Grid Interaction
Once the DC power is safely routed, the next step is to convert it into a usable form for our home’s electrical appliances, including our EV charger.
- Solar Grid Inverter: The solar grid inverter converts the DC power from the panels into alternating current (AC), which is the standard electricity form used by most household appliances including our EV charger.
- Solar Production Meter (kWh): The solar production meter tracks the amount of energy generated by our solar system and feeds it into our home’s electrical system, ensuring accurate monitoring of solar energy output.
Home Power Distribution
After conversion, the power is ready to be distributed throughout our home:
- AC Distribution Panel: The AC power flows to the AC Distribution Panel, where it’s distributed to various appliances in our home, including the EV charger. The panel contains:
- AC Surge Protector: Safeguards the system from AC voltage spikes.
- AC Disconnect Switch: A safety switch that allows for the disconnection of the AC power when needed.
- Electrical Loads or Appliances: The power is routed to our home’s appliances and, crucially, to the EV charger to charge our electric vehicle.
Grid Connection
Our solar-powered EV charging system also integrates with the external power grid to ensure a continuous power supply:
- Power Grid or Distribution Network: On the right side of the diagram, the Power Grid connects our home to the utility company’s electrical network. If the solar system is not generating enough power (e.g., at night or during cloudy weather), the home draws electricity from the grid.
- Bi-directional Solar Meter (Net Metering): The bi-directional solar meter enables net metering, measuring both electricity drawn from the grid and excess solar energy sent back. This allows us to reduce our electricity costs by receiving credits for surplus energy. With our Tesla Cybertruck and Tesla Universal Wall Connector, which supports bi-directional EV charging, our solar-powered EV charger is fully prepared for future integration. This setup not only powers our vehicle but can also send energy back to the grid or home, maximizing energy efficiency and potential savings.
- Main Service Disconnect Switch: This switch allows for the disconnection of the entire house from the grid in case of emergency or maintenance needs.
- Electrical Panel or Load Center: The main electrical panel distributes power throughout our house and to the EV charger circuit branch, ensuring a steady flow of electricity to each section.
Our Solar-Powered EV Charging System Energy Yield
Since the installation of our solar-powered EV charging system in the beginning of 2025, we have not yet gathered actual energy yield data. However, we have calculated an energy yield estimation to provide insights into the system’s performance. This estimation is essential for determining the financial viability of our system and comparing its performance to traditional energy sources.
Summary of Energy Yield Estimate
To facilitate the calculation of your solar-powered EV charging system’s energy yield, we have outlined a systematic approach that we used for estimating energy production. This approach incorporates key energy loss factors and their impact on our total power output.
The table below presents the calculation steps in a logically structured sequence based on our solar-powered EV charging system setup:
Description | Power Output (kW) |
---|---|
Nominal Power Output | 10.725 |
De-rating due to manufacturer output tolerance (5%) | 10.189 |
De-rating due to dirt (5%) | 9.680 |
De-rating due to temperature (15%) | 8.228 |
Actual DC energy after DC cable loss – PV to Inverter (3%) | 7.982 |
Actual AC energy due to Inverter efficiency (96%) | 7.663 |
Actual AC Energy after AV cable loss – Inverter to Switchboard (1%) | 7.586 |
Final Available Power Output (kW) | 7.586 |
The table provides a detailed breakdown of the solar power system’s energy output at various stages, from the nominal power to the final available power output. Initially, the system had a nominal power output of 10.725 kW. After applying various de-rating factors such as manufacturer output tolerance (5%), dirt (5%), temperature (15%), and losses due to DC cables (3%), inverter efficiency (96%), and AC cables (1%), the final available power output is 7.586 kW. This reflects the system’s real-world energy generation potential after accounting for all losses.
The energy yield calculations show that, with an average of 5 hours of peak sunlight per day, the system can produce approximately 37.93 kWh/day. Over a year, this equates to a total of 13,845.45 kWh/year of energy generation.
Factors to Consider When Estimating Solar-Powered EV Charging System Energy Yield
To accurately estimate the energy yield of our solar-powered EV charging system, we have to first calculate the nominal power output of the systems which in our case is:
Nominal Power Output = 33 panels × 325W = 10,725W (or 10.725kW)
Next, we account for power losses due to component de-rating factors and inefficiencies at various stages of the system.
Yearly Irradiation Value at the Selected Site
The amount of solar radiation received at the site throughout the year is a critical factor for estimating energy generation. This value varies based on geographical location and local weather conditions. For our site, we estimate no loss from the yearly irradiation value, assuming optimal conditions for solar generation – however, this is a factor we plan on monitoring in the future in case we experience dips in solar energy production.
De-Rating Factor for Manufacturing Tolerances
Manufacturing tolerances can slightly affect the actual power output of solar panels, which must be accounted for in the yield calculations. While these factors are not influenced by panel size, they result in minor variations from the rated output power. For our solar-powered EV charging system, we estimate a 5% loss from the rated output power due to manufacturing tolerances.
Manufacturer output tolerance = 10.725 (starting value) × (1 – 0.05) = 10.189 kW.
De-Rating Factor for Dirt
Dirt and dust accumulation on the panels reduce their efficiency by obstructing sunlight. Although dirt buildup is not affected by the panel size, it must still be factored into the energy yield calculations. For our system, we estimate a 5% loss in efficiency due to dirt accumulation on the panels.
Dirt de-rating = 10.189 × (1 – 0.05) = 9.680 kW.
Temperature De-Rating Factors
Solar panel performance is affected by temperature fluctuations, requiring a de-rating factor to account for efficiency losses as temperatures rise above the nominal 25°C. In our system, we are using polycrystalline modules, which have a typical temperature coefficient of -0.5%/°C above 25°C. Given that we expect a temperature increase of 30°C, the estimated temperature-related loss is calculated as:
30°C × 0.5% = 15% loss in efficiency
Since the estimated 15% loss due to temperature increase, the power after temperature loss would be:
Temperature de-rating = 9.680 × (1 – 0.15) = 8.228 kW.
Subsystem Efficiency (Cabling) Between PV Array and Inverter
Energy losses occur due to resistance in the cables connecting the solar panels to the inverter. Even with high-quality DC cables, we estimate a 3% loss in energy between the PV array and the inverter based on the system’s design and configuration.
DC cable loss = 8.228 × (1 – 0.03) = 7.982 kW.
Inverter Efficiency
The inverter, responsible for converting the DC electricity generated by the solar panels into AC electricity for the EV charging system, has an efficiency that impacts the overall energy yield. We have selected a high-efficiency inverter for our system, and we estimate an inverter efficiency of 96%. Consequently, we calculate the system’s output energy after accounting for inverter losses.
Inverter efficiency = 7.982 × 0.96 = 7.663 kW.
Subsystem Efficiency (Cabling) Between Inverter and Switchboard
Similar to the previous subsystem, the cables connecting the inverter to the switchboard also introduce energy losses. Given that we are using high-quality AC cables, we estimate a 1% loss in energy delivered to the switchboard from the inverter.
AC cable loss = 7.663 × (1 – 0.01) = 7.586 kW.
Final Energy Yield Estimate (in kWh)
The solar power system, with a nominal capacity of 10.725 kW, undergoes several de-rating factors, including manufacturer output tolerance (5%), dirt accumulation (5%), temperature effects (15%), DC cable losses (3%), inverter efficiency (96%), and AC cable losses (1%). After these adjustments, the system achieves a final available power output of 7.586 kW. Considering 5 hours of peak sunlight daily, the system yields approximately 37.93 kWh/day, translating to an annual energy production of 13,845.45 kWh.
- Daily Energy Yield = 7.586kW × 5hours = 37.93kWh/day
- Annual Energy Yield = 37.93kWh/day × 365days = 13,845.45kWh/year
Financial Analysis of Our Solar-Powered EV Charging System Project
Before initiating the project, we conducted a thorough cost projection for the solar-powered EV charging system. Below is a comprehensive table outlining the various expenses associated with the installation and implementation of the solar-powered EV charging system:
List of Materials for a 10kW Solar-Powered EV Charging System | Quantity | Price Per Unit (USD) | Costs (USD) |
---|---|---|---|
Solar Panels (325W) | 33 | $250 | $8,250 |
Roof-mounted racks or ground-mounted system | 33 | $100 | $3,300 |
Grid-tied inverter with MPPT (Maximum Power Point Tracking) technology | 1 | $1,200 | $1,200 |
DC Distribution Board | 1 | $500 | $500 |
AC Distribution Board | 1 | $500 | $500 |
6 AWG Copper XLPE Solar Cable | 50M | $2.50 per meter | $125 |
3-Core 25mm² Armoured AC Power Cable | 50M | $3.00 per meter | $150 |
Grounding System Kit (Earthing System Kit, Earthing Cable, and Earthing Strip) | 1 | $300 | $300 |
Safety Components (Surge Protection Device, Miniature Circuit Breaker, DC Fuses, DC and AC Disconnect Switches, MC4 Connectors) | 1 | $200 | $200 |
Lightning Arrestor | 1 | $150 | $150 |
Installation Kit (Fasteners, Nuts & Bolts) | 1 | $100 | $100 |
SPD, MCB, DC & AC Isolator, MC4 Connectors | 1 | $200 | $200 |
Service Charge | – | $500 | $500 |
Electrical Work | – | $1,000 | $1,000 |
Project Management & Design | – | $1,500 | $1,500 |
Extra Miscellaneos Costs | – | $500 | $500 |
Total Costs | $17,825 |
How Much Does It Cost to Install a Solar-Powered EV Charging System?
Based on our financial analysis, installing a 10kW solar-powered EV charging system costs approximately $17,825. This includes solar panels, inverters, distribution boards, wiring, installation, project management, and miscellaneous expenses, with potential savings from financial incentives and rebates.
Financial Incentives, Rebates, and Tax Credits for Solar Installations
In the U.S., financial incentives, rebates, and tax credits can significantly reduce the upfront cost of installing a solar-powered system. These incentives vary by state and sometimes by municipality, but here are some common programs that can help offset the cost of a solar-powered EV charging system:
Federal Investment Tax Credit (ITC)
- The Federal Solar Investment Tax Credit (ITC) allows homeowners and businesses to deduct a percentage of the cost of installing a solar energy system from their federal taxes. As of 2025, the ITC is 30% for both residential and commercial installations. This means if you spend $10,000 on a solar system, you could receive a $3,000 tax credit.
- Eligibility: You must own the system (i.e., it cannot be leased), and the system must be installed and operational during the tax year for which you’re claiming the credit.
State-Level Incentives
- Many states offer additional rebates, tax credits, or incentives to further reduce the cost of solar installations. For example:
- California offers the Self-Generation Incentive Program (SGIP), which provides rebates for installing solar and energy storage systems.
- New York offers the New York State Solar Energy System Tax Credit, which provides a state tax credit of up to 25% of the cost of a system, with a maximum of $5,000.
- Florida has a sales tax exemption for solar equipment and offers a property tax exemption for residential solar systems.
- These incentives are typically available for both residential and commercial solar installations.
Rebates from Utilities
- Some utility companies offer rebates to encourage homeowners to install solar systems. These rebates can vary greatly depending on the utility provider and geographic location. Utilities may provide a one-time rebate based on the size of the system or the amount of electricity it will generate.
- For example, Pacific Gas and Electric (PG&E) in California offers rebates for solar systems that are part of their Solar Rebate Program.
Solar Renewable Energy Certificates (SRECs)
- Some states operate Solar Renewable Energy Credit (SREC) programs. SRECs allow you to earn credits for the electricity your solar system generates. These credits can be sold to utilities or other companies that need to meet renewable energy standards.
- The amount you can earn depends on the amount of energy your solar system produces and the current market value of SRECs in your state.
Local Incentives and Property Tax Exemptions
- Some local governments offer additional incentives or rebates, which can be a combination of tax credits, cash rebates, or other financial programs.
- Several states also provide property tax exemptions for the value added by the solar system. This means that the increase in property value due to your solar installation will not be taxed.
Financing Programs
- In addition to direct rebates and tax credits, various solar financing options can help make solar installations more affordable. These include:
- Solar loans: Low-interest loans for purchasing solar systems.
- Property-Assessed Clean Energy (PACE) Financing: Allows homeowners to finance their solar installations through property taxes.
- Leases and Power Purchase Agreements (PPAs): These allow you to install solar with no upfront cost, though you pay for the energy produced over time.
How These Incentives Affect Your Solar-Powered EV Charging System Installation
For a 10kW solar-powered EV charging system, these financial incentives could reduce your overall costs by several thousand dollars. For instance, with the Federal ITC alone, we were able to reduce the system cost by 30%. State and utility rebates could add additional savings, bringing down the overall investment.
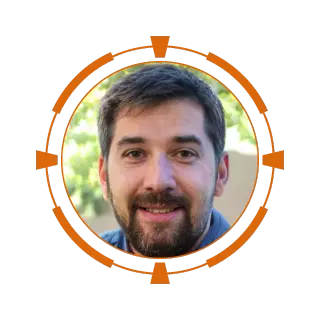
James Ndungu is a certified EV charger installer with over five years of experience in Electric Vehicle Supply Equipment (EVSE) selection, permitting, and installation. He holds advanced certifications, including the Electric Vehicle Infrastructure Training Program (EVITP), and diplomas in Electric Vehicle Technology and Engineering Fundamentals of EVs. Since 2021, James has been a frequent tester of EV chargers and accessories, providing expert insights into the latest EV charging solutions.